Science and humanities during infancy and adolescence
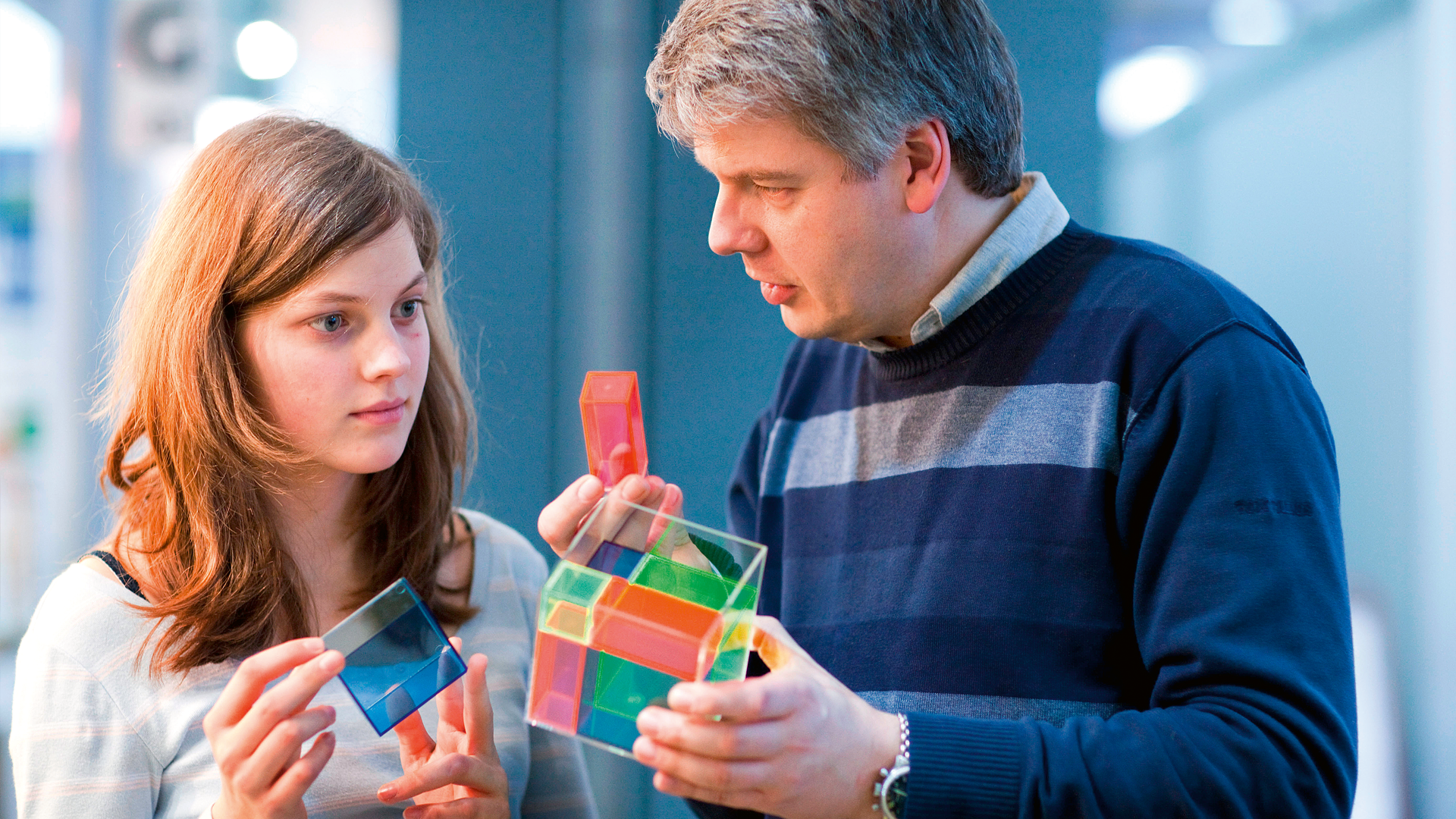
Executive summary
- Many of the world’s education systems focus on vocational specialization during secondary school. One of the principal consequences of this is that students tend to be diverted, in an almost Cartesian manner, from humanistic activities or, alternatively, from scientific activities.
- For humans, learning from the surrounding environment is a biological instinct that can be culturally exploited. For example, a toddler’s brain is wired to learn a language by imitation, trial and error, but the learning of one language or another, or deciding to learn Chinese or Russian as a second language at secondary school, is cultural.
- The main pressure acting on learning capacities and cognitive control is the need to adapt behavior to changing environmental conditions and to anticipate the uncertainties associated with these changes, which involves reasoning capacities and reflective human minds.
- Innate philosophical reasoning processes and the use of the scientific method are basic ways to acquire knowledge from early childhood, which indicates that they are an integral part of the human mind. However, as with all innate capacities, to take maximum advantage of them through life these capabilities should be nurtured throughout scholarship.
- Adolescence is a period of dramatic neural reorganization in the brain, affecting executive functions and social cognition among other processes. The way the various neural circuits mature during adolescence depends, to a certain degree, on individual experiences and how they are handled.
- It can be hypothesized that a richer educational experience—e.g., schooling that synergistically integrates the humanities and sciences through thought, reasoning, and emotions—may help generate more pluralistic and reflective human minds and alumni who are better able to self-direct their lives. This hypothesis, and the testing of it, may guide future research in educational neuroscience.
Science and humanities at secondary school: Cartesian dualism
One of the principal aims of higher education, both at universities and vocational institutions, is professional specialization. To reach this goal, education systems in much of the world, especially at secondary school, tend to focus on the adaptive and problem-solving aspects of learning, with a special emphasis on vocational specialization.1 One of the main consequences of this educational policy is that either humanistic or scientific activities are sidelined at too young an age—usually in mid-adolescence, depending on the orientation of the studies the students choose (scientific, technical, humanistic or artistic).
This educational stage coincides with a period in which the brain is undergoing dramatic neural reorganization by means of synaptogenesis, axonal sprouting, dendritic remodeling, and long-term potentiation,2 which depend to a certain degree on individual experiences and how they are handled, including learning through education. (These processes take place during all other periods of development as well.) These facts call into question the expedience of sidelining either humanistic or scientific activities at a time when providing students with as rich and varied a learning environment as possible will best contribute to the maturation of their cognitive abilities, including their executive and social cognition functions.
This is not to deny the potential benefits of some degree of specialization at secondary school that favors students’ preferences and interests but to caution against an excessive sidelining of either type of activity at an age when executive functions, including reasoning capacities, are maturing. Both humanistic and scientific activities can contribute to these development processes through their complementary viewpoints and mental strategies (as will be discussed later).
The arguments most commonly used to justify this sidelining of certain activities for secondary-school students are that in a complex world with a lot of different disciplines and professions, students need to start specializing before accessing higher education, and, equally importantly, that humanistic activities such as philosophical reasoning, as well as the scientific method, need a relatively high degree of specialization to be understood in depth and used properly.1 Philosophical reasoning can be defined as reasoned argument derived from logical thought. Similarly, the scientific method is an empirical method of acquiring knowledge through systematic observation, measurement, and experiment and through the formulation, testing, and modification of hypotheses. In some respects, despite differences in formal academic features, both philosophical reasoning and the scientific method use logical thinking. This may be a leverage point for educators, highlighting similarities (grouping) rather than differences (splitting).
Another rationale according to which philosophical reasoning or the scientific method may be sidelined is that they are both often seen, to some degree, as cultural constructs, for example, in constructivist epistemology (which has been widely discussed).3–7 According to this point of view, both philosophical reasoning and the scientific method may be learned only at the appropriate age, that is, when the brain and the cognitive processes arising from its function become sufficiently mature. This is usually considered to happen during adolescence (despite the fact that not all children develop along the exact same timetable) and coincides with the dualistic sidelining of certain activities for students. That is not to say that constructivist epistemology promotes this sidelining.
As we will see below, there is actually no “appropriate age” for learning in this sense. The cognitive skills involved in philosophical reasoning and the scientific method have long developmental time courses; even infants have rudimentary understandings of logic. In this regard, it is important to note that most current pedagogical methodologies take advantage of that and nurture philosophical and scientific reasoning during infancy and primary school, but, as this brief is intended for worldwide use, it is worth emphasizing this issue.
The following sections examine the existence from early childhood of innate philosophical reasoning processes and the innate capacity to use the scientific method to acquire knowledge from the environment and to anticipate future situations. I will also discuss the importance and significance of these innate behaviors in the context of education, with a special emphasis on the putative consequences for brain maturation of sidelining either humanistic or scientific activities at too young an age, in mid-adolescence, when the brain is being extensively rewired thus affecting behavior. Some of the ideas that will be proposed and some of the issues that will be discussed in this brief are hypotheses arising from current knowledge, so that this brief provides not only scientific data believed to be useful for educators and policymakers but also new ideas that may help to guide future research in educational neuroscience.
The instinct for learning
For humans, as well as for other animal species such as birds and mammals, learning is a basic instinct.8, 9 One of the main differences between humans and most other species is that we are able to learn throughout our lifespan, and we have the cognitive capacity to be aware of what we have learned and to use it according to previous planning,8 making use of the so-called executive functions, especially through flexibility and the ability to adapt to changing environmental contingencies and task demands.10 Executive functions are a set of cognitive processes necessary for the cognitive control of behavior. They include basic cognitive processes such as attentional control, cognitive inhibition, inhibitory control, working memory, and cognitive flexibility, and they allow the selection and successful monitoring of behaviors that facilitate reasoning, problem-solving, and the attainment of chosen goals,11 for example, learning other languages. Of course, another main difference between humans and other species is that we have culturally established schools as institutions to further learning, and, unlike other species, we count on people outside our family groups, i.e., teachers, to do the majority of the teaching of our young.
Throughout human evolution, the main selective pressure acting on learning capacities and cognitive control in the human lineage is considered to have been the need to dynamically adapt behavior to changing environmental conditions and to anticipate the uncertainties associated with these changes12 to protect ourselves from threats and take advantage of new opportunities. This combination of general processes—both those deriving from the capacity for learning and those arising from executive functions, which are intertwined—implies that philosophical reasoning and the scientific method are innate activities for the human species, which uses them from early childhood.
This fact may conflict with the view of educational policy systems1 that both philosophical reasoning and the scientific method have to be learned at the appropriate age, when the associated cognitive processes are sufficiently mature. Although infants possess learning mechanisms that do not require hypothetical thought—for example, the tracking of statistical regularities13 (i.e., that random events exhibit regularity with enough repetition, or that enough sufficiently similar random events exhibit regularity)—flexible and productive hypothesis-testing also begins in infancy. Toddlers can generate hypotheses about uncertain future events,14 flexibly adapting them to novel elements of a situation.15,16 For example, if they have a bowl of sweets wrapped in pink or yellow wrappers, and each day Mum takes a pink one and Dad a yellow one, they form the intuition that Mum definitely prefers the pink ones and Dad the yellow ones, thus anticipating future choices. There are several studies offering convincing evidence that infants are also able to measure supporting evidence17 and to test alternative hypotheses when violations occur (for example, on the day that Mum takes a yellow sweet because the bowl has run out of pink ones).18, 19
Philosophical reasoning and the use of the scientific method as innate activities during early childhood
It has been demonstrated that children naturally use the scientific method as a way of acquiring knowledge from the environment with which to anticipate future situations.20–23 And it has been shown that images induce smaller responses in the primary visual cortex of the brain when they are predictable.22 The primary visual cortex, located in the posterior pole of the occipital lobe, specializes in processing information about static objects, as well as in pattern recognition. The fact that the images induce smaller responses when they are predictable suggests that the brain does not just sit and wait for visual signals to arrive. Instead, it actively tries to predict these signals, and, when it is correct, it is rewarded by being able to respond more efficiently. If it is wrong, massive responses are required to find out why and to come up with better predictions. According to some commentary on these experiments, from the scientific point of view24 this result is strikingly similar to the job of scientists because it involves formulating a hypothesis and testing whether this hypothesis is compatible with the observations.
More specifically, the strongest evidence that children understand some of the formal principles underlying experimental design comes from research looking at children’s causal reasoning. Studies suggest, for example, that preschoolers understand patterns of covariation well enough to distinguish genuine causes from spurious associations. If two variables together generate an effect but only one of them is able to generate the effect independently, children from 2 to 4 years old conclude that the other variable is not a cause.20 Moreover, preschooler toddlers can draw accurate inferences not only from observed evidence but also from evidence they generate by chance in exploratory play. In this regard, children’s exploratory play is affected by the ambiguity of the evidence they observe.21 That is, given confounded or unconfounded evidence about which of two variables controls which of two effects, preschoolers selectively explore confounded evidence.25
To cite another example, it has been shown that, given ambiguous evidence, children’s exploratory play is sensitive to both selected and designed informative manipulations, which is typical of the scientific method.23 In an experiment, children were given basic information about candidate causes. Either four of four beads or two of four beads activated a toy when the beads were placed, one at a time, on top of the toy. The children were then shown two pairs of beads that also activated the toy. One of the bead pairs could easily be pulled apart into two individual beads, while the other pair was glued together. Although in principle only one bead in each pair might be causally effective, children explored all possible situations by themselves, pulling apart the pair that could be separated into two individual beads as well as holding the two beads that were glued together in a vertical position so that only one of the two touched the toy, and then alternating to the other. This suggests, to the extent that children acquire causal knowledge through exploration, they bridge the gap between scientific inquiry and play by understanding the utility of isolating variables, as per the scientific method.
It has also been demonstrated that the brain naturally uses philosophical-reasoning tools such as disjunctive syllogism to interpret and learn from the environment so as to anticipate future uncertainties.26, 27 Disjunctive syllogism is a valid rule of inference by which if we are told that at least one of two statements is true and also that it is not the first we can infer that it is the second statement that is true. In other words, if we are told that either A or B is true and we cannot demonstrate which is true, but we are able to demonstrate that, for example, A is false, then we necessarily infer that B is true.
This reasoning capacity was initially reported in toddlers using the “cups task”,28 in which toddlers were shown two cups—one empty and the other containing a reward. Investigators evaluated how often, when later shown the empty cup again, the toddlers went directly to the cup containing the reward, which implied the use of inference based on disjunctive syllogism26 to anticipate an uncertain situation from previous learning. In this experiment, applied to children from 23 months to 5 years old, two stickers were hidden inside two pairs of cups, with one sticker in one cup of each pair. One cup of one of the pairs was then revealed to be empty. If children were reasoning using disjunctive syllogism, they could combine this information (not A of pair 1) with their representation of where the sticker was hidden (A or B in pair 1) to conclude that the cup paired with the empty one necessarily contained a sticker (therefore B), while the location of the other sticker (in one of the cups of pair 2) was unsure. According to the conclusions drawn by the authors of this experiment, 3- to 5-year-olds used disjunctive syllogism properly while toddlers younger than 2.5 years old did not.
However, other experiments with a simpler design show that preverbal infants may use precursors of logical reasoning in an innate manner.27 Infants aged between 12 and 19 months old were presented with ambiguous scenarios about the identity of an object, which could be resolved through inference derived from disjunctive syllogism. Two puppets, differing in shape, texture, color, and category but with identical top parts—for example, a dinosaur and a flower with the same plume-shape structure at the top—enter a theater. An occluder hides them, and then a cup scoops one of them from behind it, with only the top part visible. Thus, the infants cannot know the identity of the scooped object and may establish a disjunctive representation. Then the occluder moves downward, revealing one whole object—for example, the dinosaur—so the infants have evidence with which to disambiguate the identity of the scooped object by disjunctive syllogism. Lastly, the dinosaur leaves the stage, and the cup reveals the second object: that is, the flower.
What is crucial in this experiment is that half the time the revealed object is consistent with the conclusion suggested by logical inference (that is, the flower), but for the other half it is inconsistent, and the revealed object, which has been manipulated by the investigators, is, surprisingly for participants, the dinosaur. Experimenters observed the eyes of the infants in each of these phases. Both the 12-month-olds and 19-month-olds spent longer looking at the inconsistent outcome, and their pupils dilated, suggesting that they may have derived the identity of the object in the cup through logical inference and were surprised when this conclusion was violated. These oculomotor markers resembled those of adults inspecting similar scenarios, suggesting that intuitive and stable logical structures involved in the interpretation of dynamic scenes may be part of the fabric of the human mind. In other words, at least some philosophical-reasoning tools, such as disjunctive syllogism, seem to be innate in our species from early childhood, implying the use of the scientific method to acquire knowledge from the environment with the aim of anticipating future uncertainties.
Coming back to the scaffold of this brief, as the capacity to learn from the environment to anticipate future uncertainties is maintained throughout life, and taking into account that adolescence is a period of extensive neuronal reorganization in the brain when crucial cognitive processes such as executive functions mature, the relevant question here is why these innate (but educationally augmentable) processes of philosophical reasoning and scientific method have to be sidelined at secondary school, or to what extent they should be sidelined to favor a particular specialization. In other words, what might be the consequences for the development and maturation of the brain if one of these two complementary ways of acquiring useful knowledge is sidelined during adolescence?
Putative consequences of excessive dualistic sidelining of either humanistic or scientific activities for brain maturation: A hypothesis
Adolescence is a period of dramatic neural reorganization in the brain. The maturation of various neural circuits during this stage of development depends, to a large degree, on individual experiences and how they are handled, as has been demonstrated (to cite a couple of examples) in relation to the digital era29 or resilience modeling.30 For example, one review suggested that media use, as processed by the developing adolescent brain, may contribute to sensitivity to online rejection, acceptance, peer influence and emotion-laden interactions in media environments.29 As a result, it has been suggested that peer sensitivities are possibly greater in adolescents than in older age groups. This is especially relevant, for example, when considering the effect of peer influence that encourages adolescents to take more risks in the presence of their peers because peers can likewise stimulate risk-taking through online comments. Further, it has been speculated30 that the ongoing development of social regions of the brain combined with a strong sensitivity to acceptance and rejection may make adolescence particularly vulnerable to social media on their self-image and expectations of self and others. Furthermore, the emerging trajectory of acceptance sensitivity, peer obedience and emotional precedence may make adolescents more susceptible to sensationalist and fake news or unrealistic self-expectations and less adept at regulating emotions, including resilience.30
Negative social experiences during adolescence have been reported as being key factors in several stress-related mental illnesses.31 It has been shown, using rats in experimental system modeling, that social play-fighting behavior during early adolescence is essential for the final maturation of brain and behavior, and that manipulation of the rat adolescent social experience alters many neurobehavioral measurements implicated in anxiety, depression and substance abuse. In humans, social stress during adolescence also leads to the development of anxiety and depressive behavior as well as escalated drug use in adulthood, altering stress-related neural circuits and the associated neurotransmitters (such as those of the monoaminergic system).
Neural reorganization occurs through a process of neural plasticity, which, against a backdrop of physical and functional structures, adapts the nervous system to environmental demands, physiological changes, or new experiences. These structures depend on both genetic and epigenetic factors as well as on previous experiences. Plasticity during adolescence entails an alteration of the connections between neurons through different mechanisms, including axonal sprouting, dendritic remodeling, synaptogenesis, and long-term potentiation—all of which alter synaptic efficacy.2 Social, emotional, and cognitive experiences, including those afforded by learning at secondary school, may affect the structure and function of the brain’s neural networks, subserving different domains of behavior. Although all of these processes occur throughout childhood, during adolescence the networks related to social cognition and executive functions mature: reasoning capacity, decision-making, attentional control, cognitive inhibition, inhibitory control, working memory, and cognitive flexibility, among others, allowing the selection and successful monitoring of behaviors that facilitate the attainment of chosen goals and directed problem-solving.
The educational policy mentioned above, which favors specialization, tends to sideline either humanistic or scientific activities for adolescent students. Both of these activities are used in an innate manner from early childhood, and it could be suggested that this may be detrimental to students’ ability to be critical and to solve problems more globally by integrating different perspectives, approaches, and points of view. This includes scientific and technical factors, as well as humanistic ones such as ethical and social considerations. This policy could affect the ability to self-direct one’s own life because it might limit the person’s ability to predict and prevent problems, and it may also influence individual behavior by affecting the ongoing construction of the brain32–34 in a period in which this organ is being extensively rewired. This hypothesis remains to be confirmed or dismissed, but it may serve to direct further research in educational neuroscience.
All learning, at every educational level, whether of concepts (regardless of whether these are humanistic, scientific or technological), skills (procedural learning), or attitudes (inclusiveness, respect, critical and reflective assessment of situations, dialogue-seeking to resolve conflicts, empowerment of one’s own life history, etc.) is stored in the brain as memory in the form of patterns of neural connections. Learning fuels the brain, and this conditions a person’s self-image as well as their view of their environment and how they relate to it.35 An education that synergistically and harmoniously integrates the humanities and sciences through thought, reasoning, and emotions may contribute to generating more pluralist and reflective human minds. To put it bluntly, secondary-level education that integrates humanistic, artistic, scientific, and technological knowledge in a dynamic way—by using their epistemological particularities to address different issues from all possible angles—may help forge the next generation of adults to have a greater mental capacity to integrate, value, and reflect on any situation, professionally and personally.1 A possible outcome would be individuals who have a greater capacity to contemplate and appreciate situations by themselves, based on the information around them, who become involved in the search for solutions (like those, for example, made explicit in the UN Sustainable Development Goals) and who commit to making those solutions happen, both individually and collectively.36
References
- Bueno, D., Casanovas, J., Garcés, M., & Vilalta, J. M. (Eds.) (2019). Higher education in the world 7: Humanities and higher education—Synergies between science, technology and humanities. Barcelona: Global University Network for Innovation (GUNi).
- Fandakova, Y. (2020). Mechanisms of learning and plasticity in childhood and adolescence. Developmental Cognitive Neuroscience, 42, 100764.
- Piaget, J. (Ed.). (1967). Logique et connaissance scientifique. In Encyclopédie de la Pléiade, vol. 22. Paris: Éditions Gallimard.
- Watzlawick, P. (1984). The invented reality: How do we know what we believe we know? Contributions to constructivism. New York, NY: W. W. Norton.
- Suchting, W. A. (1992). Constructivism deconstructed. Science & Education, 1(3), 223–54.
- Slezak, P. (2000). A critique of radical social constructivism. In D. C. Philips (Ed.), Constructivism in education: Opinions and second opinions on controversial issues (pp 91–126). Chicago, IL: National Society for the Study of Education.
- Rockmore, T. (2008). On constructivist epistemology. Lanham, MD: Rowman & Littlefield.
- Gould, J. L. (2004). Animal cognition. Current Biology, 14(10), R372–R375.
- Marler, P. (2004). Innateness and the instinct to learn. Anais da Academia Brasileira de Ciências, 76(2), 189–200.
- Fleming, K. A., Heintzelman, S. J., & Bartholow, B. D. (2016). Specifying associations between conscientiousness and executive functioning: Mental set shifting, not prepotent response inhibition or working memory updating. Journal of Personality, 84(3), 348–360.
- Diamond, A. (2013). Executive functions. Annual Review of Psychology, 64, 135–68.
- Teffer, K., & Semendeferi, K. (2012). Human prefrontal cortex: Evolution, development, and pathology. Progress in Brain Research, 195, 191–218.
- Saffran, J. R., Aslin, R. N., & Newport, E. L. (1996). Statistical learning by 8-month-old infants. Science, 274, 1926–1928.
- Téglás, E., Girotto, V., Gonzalez, M., & Bonatti, L. L. (2007). Intuitions of probabilities shape expectations about the future at 12 months and beyond. Proceedings of the National Academy of Sciences of the United States of America,104, 19156–19159.
- Téglás, E., Vul, E., Girotto, V., Gonzalez, M., Tenenbaum, J. B., & Bonatti, L. L. (2011). Pure reasoning in 12-month-old infants as probabilistic inference. Science, 332(6033), 1054–1059.
- Téglás, E., Ibanez-Lillo, A., Costa, A., & Bonatti, L. L. (2015). Numerical representations and intuitions of probabilities at 12 months. Developmental Science, 18, 183–193.
- Gweon, H., Tenenbaum, J. B., & Schulz, L. E. (2010). Infants consider both the sample and the sampling process in inductive generalization. Proceedings of the National Academy of Sciences of the United States of America, 107, 9066–9071.
- Gweon, H., & Schulz, L. (2011). 16-month-olds rationally infer causes of failed actions. Science, 332, 1524.
- Stahl, A. E., & Feigenson, L. (2015). Cognitive development: Observing the unexpected enhances infants’ learning and exploration. Science, 348(6230), 91–94.
- Gopnik, A., Sobel, D. M., Schulz, L. E., & Glymour, C. (2001). Causal learning mechanisms in very young children: Two-, three-, and four-year-olds infer causal relations from patterns of variation and covariation. Developmental Psychology, 37(5), 620–629.
- Schulz, L. E., & Bonawitz, E. B. (2007). Serious fun: Preschoolers engage in more exploratory play when evidence is confounded. Developmental Psychology, 43(4), 1045–1050.
- Alink, A., Schwiedrzik, C. M., Kohler, A., Singer, W., & Muckli, L. (2010). Stimulus predictability reduces responses in primary visual cortex. Journal of Neuroscience, 30(8), 2960–66.
- C. Cook, C., Goodman, N. D., & Schulz, L. E. (2011). Where science starts: Spontaneous experiments in preschoolers’ exploratory play. Cognition, 120(3), 341–349.
- Singer, W. (2010, March 10). The scientific brain: The human brain processes predictable sensory input in a particularly efficient manner. Max-Planck-Gesellschaft. https://www.mpg.de/619356/pressRelease201003101
- Gopnik, A., Meltzoff, A. N., & Kuhl, P. K. (2000). The scientist in the crib: What early learning tells us about the mind. New York, NY: HarperCollins.
- Mody, S., & Carey, S. (2016). The emergence of reasoning by the disjunctive syllogism in early childhood. Cognition,154, 40–48.
- Cesana-Arlotti, N, Martín, A., Téglás, E., Vorobyova, L., Cetnarski, R., & Bonatti, L. L. (2018). Precursors of logical reasoning in preverbal human infants. Science, 359(6381), 1263–1266.
- Hill, A., Collier-Baker, E., & Suddendorf, T. (2011). Inferential reasoning by exclusion in great apes, lesser apes, and spider monkeys. Journal of Comparative Psychology, 125, 91–103.
- Crone, E. A., & Konijn, E. A. (2018). Media use and brain development during adolescence. Nature Communications, 9, 588.
- Malhi, G. S., Das, P., Bell, E., Mattingly, G., & Mannie, Z. (2019). Modelling resilience in adolescence and adversity: A novel framework to inform research and practice. Translational Psychiatry, 9, 316.
- Burke, A. R., McCormick, C. M., Pellis, S. M., & Lukkes, J. L. (2017). Impact of adolescent social experiences on behavior and neural circuits implicated in mental illnesses. Neuroscience & Biobehavioral Reviews, 76(B), 280–300.
- Tong, D., Lu, P., Li, W., Yang, W., Yang, Y., Yang, D., Qiu, J., & Zhang, Q. (2019). Critical thinking and regional gray matter volume interact to predict representation connection in scientific problem solving. Experimental Brain Research, 237(8), 2035–2044.
- Takeuchi, T., Duszkiewicz, A. J., & Morris, R. G. (2013). The synaptic plasticity and memory hypothesis: Encoding, storage and persistence. Philosophical Transactions of the Royal Society B, 369, 20130288.
- Sweatt, J. D. (2016). Neural plasticity and behavior: Sixty years of conceptual advances. Journal of Neurochemistry,139(suppl. 2), 179–199.
- Cortese, A., Amano, K., Koizumi, A., Kawato, M., & Lau, H. (2016). Multivoxel neurofeedback selectively modulates confidence without changing perceptual performance. Nature Communications, 7, 13669.
- UN General Assembly. (2015). Transforming our world: The 2030 agenda for sustainable development. A/RES/70/1.