Epigenetics and learning: How the environment shapes gene expression, and the possible consequences for learning and behaviour
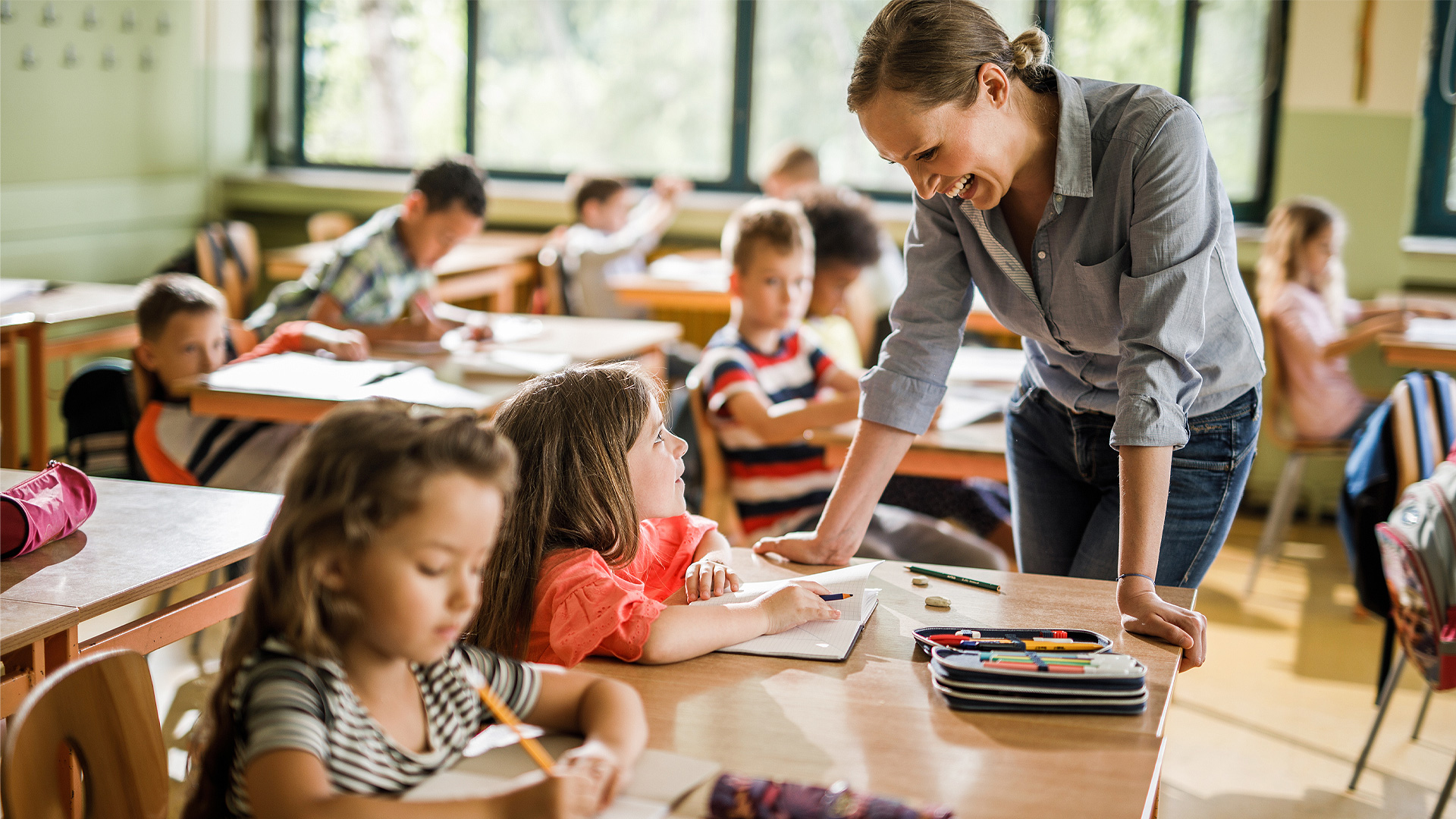
Executive Summary
- Genes shape the brain and affect various cognitive functions, such as learning processes, through a complex interaction with multiple environmental factors.
- Several mechanisms regulate gene expression, such as epigenetic modifications that link environmental cues to the genetic machinery. Epigenetic signatures consist in the controlled addition of specific molecules to the genetic material of cells that do not change the DNA sequence but that rather can affect gene activity.
- Childhood experiences to the generation of epigenetic signatures that facilitate neural plasticity, that is the ability of neural networks in the brain to change through growth and reorganization, and thus further learning processes.
- Adverse childhood environmental conditions, such as those associated with negative parenting or trauma, contribute to the establishment of epigenetic modifications that may impair cognitive function necessary for learning and building a stable personality, although having even one dependable, caring, supportive adult in a child’s life, as for example a teacher, can help to counteract the effects.
- Adolescent lifestyles, especially where there is drug consumption, may negatively alter the epigenome of gametes and affect future offspring.
It is important to share this knowledge among not only educational professionals, policy makers and families, but also among adolescents who will generate the families of the future.Biologically, a child’s learning capacity allows behavioural responses to adapt progressively to a complex, dynamic, changing, and uncertain environment, modifying aspects that may favour the survival of the individual. The mental functions that impact all aspects of human behaviour, including learning, arise from brain activity. Neural connections are formed throughout life to generate and support mental function, and thereby, enable lifelong learning. Brain formation and function, as well as neural plasticity, are ultimately influenced by gene activity and by epigenetic modifications, with the latter offering a crucial regulation of gene expression in adaptation to environmental conditions. In other words, how the brain grows, maturates and functions is influenced by both genes and environment, and also by the specific interaction between genes and environment. The environment –which includes familial, social and educational environments, as well as all experiences a person has through his/her life– can affect neural connectivity and can also affect genes’ activity, turning them on and off, through epigenetic modification.In this brief, the role of epigenetic contributions to the mental aspects of learning will be discussed, touching on the genome and its influence on brain construction and cognitive function, as well as how gene expression is regulated. I aim to enhance, among education professionals, parents, adolescents, and policy makers, understanding of these biological origins of differences in mental capabilities as mediated by the environment. Given that these differences arise in familial, social, and educational environments, I anticipate that these groups will benefit from being empowered with the knowledge to adopt more respectful and flexible educational practices.
A Taste of Genetics: How Genes Help to Shape the Brain
The brain forms during embryonic and fetal development under the direction of certain genetic programmes1 and continues to build and rebuild its connectome (i.e., the map of neural connections) throughout life. This process of neuronal plasticity, which has been discussed in other briefs in this series, forms the cellular basis of learning. The ability to learn also requires many different cognitive functions, such as working memory, attention, intelligence, cognitive control, and executive functions, as well as related functions, such as motivation and resilience. These functions arise through the brain activity, which in turn relies on its development and the formation of the connectome. In this context, both the construction of the brain and the functioning of its neurons depend partially on genetic programmes.
The human genome comprises some 20,300 genes2, all of which may present several alleles (i.e., genetic variants that differ in their nucleotide sequence). A gene may be defined as a unit of heredity that is transferred from a parent to offspring and that determines a biological characteristic. Each gene consists of a distinct sequence of nucleotides, the basic molecules that constitute DNA, with the order of these encoding specific biological information. Slight differences in the nucleotide sequences of alleles may therefore alter that encoded information, which in turn, may be reflected in the biological functions they control or may influence the synthesis of corresponding proteins. This control due to the genetic influences of distinct alleles can be far-reaching. Indeed, just as people may have individual variations in physical traits (e.g., different blood types, features, and heights) they may also show variations in psychological traits, including those associated with cognitive function and learning capacity. For example, it has been reported that 800 genes exist with different alleles that may affect to some extent general cognitive function or temperament3-5, which do not which does not exclude the important influence of environmental factors.
Despite the existence of genetic influences on all cognitive functions associated with learning, there is no doubt that certain factors in aspects related to mental life can adversely affect the influence of each gene or allele. These factors include (1) the high number of genes and alleles involved; (2) the usually complex interactions among them; (3) the fact that any gene or allele may influence different psychological domains; and (4) the influence of familial, social, and educational environments on mental function. Consequently, the most informative data from an educational perspective arise from so-called heritability, a statistic that is typically interpreted as capturing how much of the variation of a trait is due to genetic differences. Technically, heritability could be defined as the proportion of variability in any observable characteristic associated with genetic variation in a population6,7 and expressed on a scale ranging from 0 to 1 (or percentages). However, variations in heritability are best interpreted as percentages, with genetic and environmental effects required to equal 100% (or 1.0 as unitary trait). Using such an approach, it is possible to see that any increase in environmental contribution to the variance of the trait analysed implies a corresponding decrease in the relative genetic contribution, and vice versa.
An increasing number of papers have been published in which the heritability of psychological traits and cognitive functions are studied. However, there is no universal agreement in the values reported, mainly due to the methods used. As examples, Annex 1 summarises the heritability of Holland’s Six Personality Traits, whereas Annex 2 summarises the heritability of some traits linked to learning processes. The important issue about these data is not the specific values they show, but realizing some genetic involvement in many, if not all, aspects of a person’s cognitive abilities and temperament.
The heritability of cognitive traits also emphasises the importance of environmental factors in shaping cognitive functions by affecting the brain, as experiences contribute to shape neural networks (the connectome) by means of neural plasticity. For example, it is known the existence of substantial underlying neural plasticity associated with development that supports behavioural changes during adolescence8, as well as during childhood9. Although such neural plasticity is beyond the scope of this brief and it has been dealt in previous briefs in this series, it is important to highlight its involvement in modifying and adapting behaviour through learning.
Gene Expression is Tightly Regulated: from Enhancers and Transcription Factors to Epigenetic Modifications
Virtually all human cells contain a complete genome; however, the body possess more than 200 different cell types that have diverse morphologies and physiological functions. This simple observation highlights the importance of regulating gene expression through the wide range of mechanisms employed by cells to increase or decrease the production of specific gene products10. Sophisticated programmes of gene expression are widely observed to trigger developmental pathways or respond to environmental stimuli. Skin cells and muscle cells, for instance, differ in appearance and function because they express different genes. Moreover, within a specific cell type, some genes are only expressed under certain conditions or at certain times. An example of this is that pain due to a burn has been shown to upregulate the expression of monoamine oxidase A (MAO-A) gene in the hippocampus of mouse, leading to delayed depressive behaviour and anxiety11. MAO-A is involved in the degradation of some neurotransmitters such as dopamine, norepinephrine, and serotonin. In this regard, it is important to note that most of the manipulative experiments have been performed in rodents such as mouse and rat, as they are genetically and epigenetically very close to humans, which allows to extrapolate to a certain extent obtained data12.
Gene expression has been reported to be regulated by different systems10, being epigenetic signatures one of them (Figure 1). During the last two decades, an increasing amount of evidence has suggested that various environmental factors help to regulate gene expression by establishing specific epigenetic modifications on particular genes. This area of research, which used to be called ‘neuroepigenetics’, has emerged as an important neuroscientific domain in which environmental factors are linked to the gene functions that affect cognitive functions13. As such, neuroepigenetics has further expanded the concept of environmentally triggered neural plasticity, being considered to concern the regulation of gene expression mediated by changes in DNA methylation and chromatin structure14. It is noteworthy that in the DNA molecule, the nucleotide sequence that contains genes, is wrapped around several proteins called histones to form chromatin. Epigenetic modifications do not alter the information encoded by genes, and instead, only affect the way in which they are expressed. Although reversible, they tend to be maintained for a long time.
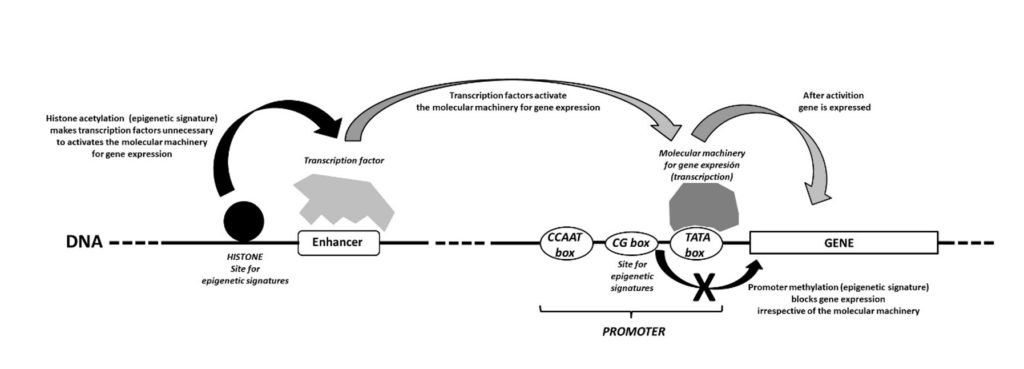
Figure 1. Regulation of gene expression by promoters, enhancers, and transcription factors. This regulatory system is highly effective, and it allows for quick responses to environmental changes. However, this is at the cost of high metabolic and energy demands. Thus, other systems are clearly needed to regulate gene expression, particularly where the expression must be regulated in the same manner for prolonged periods. It is here that epigenetic modifications step in, providing a much more cost-effective option in terms of metabolic and energy demands over the long-term. Sites for epigenetic signatures are included, and their role on gene expression is shown. Modified from [15].
There are two main types of epigenetic modification, either directly on the DNA molecule itself or on the chromatin histones14 (Figure 1). These result in complex epigenetic code modulating gene expression. Despite the biochemical complexity of these processes, there are two important take home messages for the scope of this brief: (1) epigenetic modifications are essential for adaptative long-term regulation of gene expression, and (2) some of these modifications are established by environmental influences. In other words, the study of epigenetics allows us to consider the link between environmental factors and gene function to understand how physiology and behaviour may adapt. This includes behaviours that appear causally linked with learning processes seen in a broader sense. It may also be possible for these changes to be passed to offspring, as discussed below.
Epigenetic Modifications Link the Environment with Gene Expression: Consequences for the Development of Cognitive Functions
Although the specifics of how environmental factors trigger epigenetic modifications are mechanistically and enzymatically complex, they are reasonably well-known14. Epigenetics can be defined as the study of phenomena and mechanisms that cause chromosome-associated changes to gene expression that are inherited during cell replication and that are not dependent on changes in DNA sequence. In this context, the epigenome refers to the specific pattern of epigenetic modifications present in a cell at a given time. During its life span, an individual has one genome, which can be modified at different times to produce many different epigenomic states. Unlike the genome, which is identical in all cells, the epigenome is specific to cell types and changes throughout the life cycle in response to environmental cues. In this regard, to cite an example related to educational systems, it has been suggested the existence of epigenetic processes affecting the development of cognitive abilities and learning within the educational related to stress and bullying16. In this regard, although strict education certainly succeeds in creating some kind of “excellence,” it does so at a very high cost. Many will drop out during the process, and the stayers may do so with a legacy of trauma in their brains.
Epigenetic modifications also contribute to regulating the expression of genes involved in neural plasticity and neuron physiology, including the complex networks of interactions in the neurotransmitter system. This fact emphasises the relevance of epigenetic changes for learning and mental function, including cognitive ability, in relation with environmental cues. These are especially relevant during childhood when human beings adapt more intensely to the environment in which they live and grow. It is important to note that epigenetic signatures form an adaptive system that is influenced by a range of experiences and environmental conditions, with their main biological role being to favour adaptation of the host individual.
Thus, it has been reported that memory formation and storage, and consequently learning processes, requires the epigenetic signatures of certain genes, such as those that enhancing neural plasticity17-19. In this way, engaging in learning facilitates future learning in a positive feedback loop that promotes epigenetic signatures facilitating neural plasticity. In other words, the epigenome of teenagers who have been stimulated during childhood better promotes the expression of genes that will facilitate the acquisition of new knowledge by enhancing brain plasticity. For example, using rats as model system, it has been demonstrated that environmental enrichment has have profound effects on the healthy adult brain and as a remedial tool for brains compromised by injury, disease, or negative experience20.
It has also been demonstrated that differences in epigenetic signatures influence assessments of executive function21, and that malnutrition in early childhood is associated with epigenetic modifications that can impair attention and cognition22. Similarly, epigenetic variance in the gene for the dopamine D2 receptor affects the malleability of intelligence23,24. Although neuroepigenetics is a relatively new field of research, the evidence of its importance in regulating cognitive functions is growing rapidly.
Of special interest are the effects of early childhood traumas and educational environments on cognitive development. For example, it has been reported that childhood abuse correlates with epigenetic regulation of glucocorticoid receptors in the brain, favouring the manifestation of depressive behaviour later in adolescence and adulthood25-26. In one of this works25, to analyse one example, epigenetic differences in a neuron-specific glucocorticoid receptor (NR3C1) promoter between postmortem hippocampus obtained from suicide victims with a history of childhood abuse and those from either suicide victims with no childhood abuse or controls were compared. Findings of this work may translate previous results from rat to humans, suggesting a common effect of parental care on the epigenetic regulation of hippocampal glucocorticoid receptor expression. This specifically affects the hypothalamic–pituitary–adrenal axis26, as well as the MAO-A gene, which is associated with impulsive behaviour27. Similarly, childhood neglect has been correlated with specific epigenetic signatures that have implications for psychiatric vulnerability28. Elsewhere, it has been shown that negative parenting, in which there is indifference, neglect, rejection, hostility, or little emotional warmth, correlates with epigenetic modifications in a set of genes that favour depression in later life29.
Although the examples in this text have detailed the epigenomic changes that occur in a given individual in response to adverse environmental conditions, it has also been reported that specific signatures can be passed to offspring trough gametes (spermatozoids or oocytes) in some cases. This fact appears to be especially relevant in relation to drugs consumed during adolescence. The effects of in utero exposure to illicit drugs on adult offspring are well established, particularly regarding the growing recreational use of cannabis, ketamine, and MDMA (3,4-methylenedioxymethamphetamine). Epigenetic mechanisms, including DNA methylation and histone modifications, provide a mechanistic link between the prenatal environment (i.e., drugs consumed by the pregnant mother) and the adolescent environment (i.e. drugs taken by adolescents) concerning health consequences. These may present years after the original exposure, with shifts in the epigenome in early life or adolescence having the potential to induce behavioural and somatic disease states that may only appear during adulthood30. Furthermore, it has been demonstrated that cannabis use during adolescence may trigger epigenetic signatures in gametes that can alter cognitive function in offspring that are yet to be conceived. Specific consequences include decreases in the capacity to concentrate and focus, as well as an increased risk of depression31.
Despite the fact that most of the work cited in this brief focus on negative experiences and their epigenetic effects on cognitive vulnerability, as most of the studies are centred in these issues, there are also some data concerning positive experiences, as for example those cited on positive versus negative parenting29. To cite other example, it has been demonstrated in rats that maternal care and exposure of the young pups to social behaviour could affect the epigenome of hundreds of genes in the adult hippocampus positively affecting stress response and anxiety-mediated behaviours32-34. That is, by decreasing stress and anxiety through better management of these mental processes in potentially threatening situations.
Summarizing, this adaptive system of epigenetic signatures is influenced by many different experiences and environmental conditions, without which adaptation to changing environments would be more difficult. As such, it parallels neural plasticity in modifying behaviour through learning. Given that these differences arise in familial, social, and educational environments, these groups will benefit from being empowered with the knowledge to adopt more respectful and flexible educational practices. I this regard, it is important to highlight that it has also been shown that having even one dependable, caring, supportive adult in a child’s life (e.g., a teacher) can help to counteract these effects35, which justify the essential role of educational practitioners to adapt and to make more flexible the educational practices to students’ needs.
Conclusions
In summary, several environmental factors, such as parenting, teaching methodologies, traumas, etcetera, are increasingly being recognised as important to the regulation of gene function that affects personality and cognitive function though specific epigenetic signatures. The works cited in this brief represent only the tip of a large iceberg. However, my goal was not to conduct an extensive review of studies linking epigenetics and behaviour, but rather to highlight the importance of learning environments on both neural plasticity and genomic expression through epigenetic signatures. This research therefore extends beyond the mere heritability of different learning processes and cognitive functions, and moves to include familial, social and educational environments during childhood and adolescence as crucial for not only neural plasticity but also the epigenome. And possibly may also include teaching methodologies, which taken to the ends can be strict and stressful or supportive and adjusted to diversity and to natural rhythms of brain development. In this way, the epigenome acts indirectly on cognitive processes such as learning through a variety of behaviours. Similarly, by establishing epigenetic signatures, lifestyle during adolescence appears to be crucial for the development of future offspring. This emphasises the importance of introducing educational professionals, families, and adolescents alike to the knowledge in this brief. In this way, we can have the greatest impact on the families of the future acting from the present.
References
- Brown, M., Keynes, R., & Lumsden, A. (2001). The Developing Brain. Oxford: Oxford University Press.
- Salzberg, S. L. (2018). Open questions: How many genes do we have? BMC Biology 16:94.
- Davies, G., Lam, M., et al. (2018). Study of 300,486 individuals identifies 148 independent genetic loci influencing general cognitive function. Nat. Commun. 9(1): 2098.
- Zwir, I., Arnedo, J., Del-Val ,C., Pulkki-Råback, L., Konte, B., Yang, S. S., Romero-Zaliz, R., Hintsanen, M., Cloninger, K. M., Garcia, D., Svrakic, D. M., Rozsa, S., Martinez, M., Lyytikäinen, L. P., Giegling, I., Kähönen, M., Hernandez-Cuervo, H., Seppälä, I., Raitoharju, E., de Erausquin, G. A., Raitakari, O., Rujescu, D., Postolache, T. T., Sung, J., Keltikangas-Järvinen, L., Lehtimäki, T., & Cloninger, C. R. (2020). Uncovering the complex genetics of human temperament. Mol. Psychiatry. 25(10): 2275-2294.
- Zwir, I., Arnedo, J., Del-Val, C., Pulkki-Råback, L., Konte, B., Yang, S. S., Romero-Zaliz, R., Hintsanen, M., Cloninger, K. M., Garcia, D., Svrakic, D. M., Rozsa, S., Martinez, M., Lyytikäinen, L. P., Giegling, I., Kähönen, M., Hernandez-Cuervo, H., Seppälä, I., Raitoharju, E., de Erausquin, G. A., Raitakari, O., Rujescu, D., Postolache, T. T., Sung, J., Keltikangas-Järvinen, L., Lehtimäki, & T., Cloninger. C. R. (2020). Uncovering the complex genetics of human character. Mol. Psychiatry. 25(10): 2295-2312.
- Sesardic, N. (2005). Making Sense of Heritability. Cambridge: Cambridge University Press.
- Visscher PM, Hill WG, & Wray NR. (2008). Heritability in the genomics era–concepts and misconceptions. Nat. Rev. Genet. 9(4): 255-266.
- Sachser, N., Hennessy, M. B., & Kaiser S. (2018). The adaptive shaping of social behavioural phenotypes during adolescence. Biol. Lett. 14(11).
- Miskolczi, C., Halász, J., & Mikics, É. (2019) Changes in neuroplasticity following early-life social adversities: the possible role of brain-derived neurotrophic factor. Pediatr. Res. 85(2): 225-233.
- Perdew, G.H., Vanden Heuvel, J.P., & Peters, J.M. (2006) Regulation of Gene Expression: Molecular Mechanisms. New York: Human Press.
- Wang, Z., Chen, L., Rong, X., & Wang, X. (2017). Upregulation of MAOA in the hippocampus results in delayed depressive-like behaviors in burn mice. Burns 4179(17):30165.
- Ledo, N., Phillips, H., & Roth, T.L. (2019). Animal models and their contribution to our understanding of the relationship between environments, epigenetic modifications, and behavior. Genes 10(1): 47.
- Sweatt J.D. (2013). The emerging field of neuroepigenetics. Neuron. 80(3): 624-632.
- Armstrong, L. (2019). Epigenetics. Boca Ratón: CRC Press
- Bueno, D. (2018). Epigenoma. Barcelona: Plataforma Editorial.
- Frías-Lasserre, D., Villagra, C.A., & Guerrero-Bosagna, C. (2018). Stress in the educational system as a potential source of epigenetic influences on children’s development and behavior. Front. Behav. Neurosci. 12: 143.
- Day, J. J., & Sweatt, J. D. (2010). DNA methylation and memory formation. Nat. Neurosci. 13: 1319-1323.
- [18] Collins, B. E., Greer ,C. B., Coleman, B. C., & Sweatt J. D. (2019) Histone H3 lysine K4 methylation and its role in learning and memory. Epigenetics Chromatin. 12(1): 7.
- Schmauss, C. (2017). The roles of class I histone deacetylases (HDACs) in memory, learning, and executive cognitive functions: A review. Neurosci. Biobehav. Rev. 83: 63-71.
- Mychasiuk, R., Zahir, S., Schmold, N., Ilnytskyy, S., Kovalchuk, O., & Gibb, R. (2012). Parental enrichment and offspring development: modifications to brain, behavior and the epigenome. Behav. Brain Res. 228(2): 294-298.
- Ibrahim, O., Sutherland, H. G., Haupt, L. M., & Griffiths, L. R. (2018). An emerging role for epigenetic factors in relation to executive function. Brief Funct. Genomics. 17(3): 170-180.
- Peter, C. J., Fischer, L. K., Kundakovic, M., Garg, P., Jakovcevski, M., Dincer, A., Amaral, A. C., Ginns, E. I., Galdzicka, M., Bryce, C. P., Ratner, C., Waber, D. P., Mokler, D., Medford, G., Champagne, F. A., Rosene, D. L., McGaughy, J. A., Sharp, A. J., Galler, J. R., & Akbarian, S. (2016). DNA Methylation signatures of early childhood malnutrition associated with impairments in attention and cognition. Biol. Psychiatry. 80(10): 765-774.
- Delvecchio, G., Bellani, M., Altamura, A. C., & Brambilla, P. (2016). The association between the serotonin and dopamine neurotransmitters and personality traits. Epidemiol. Psychiatr. Sci. 25(2): 109-112.
- Kaminski, J., Schlagenhauf, F., Rapp, M., Awasthi, S., Ruggeri, B., Deserno, L., Banaschewski, T., Bokde, A. L. W., Bromberg, U., Büchel, C., Quinlan, E. B., Desrivières, S., Flor, H., Frouin, V., Garavan, H., Gowland, P., Ittermann, B., Martinot, J. L., Martinot, M. P., Nees, F., Orfanos, D. P., Paus, T., Poustka, L., Smolka, M. N., Fröhner, J. H., Walter ,H., Whelan, R., Ripke, S., Schumann, G., & Heinz, A. (2018) Epigenetic variance in dopamine D2 receptor: a marker of IQ malleability? Transl. Psychiatry. 8(1): 169.
- McGowan, P. O., Sasaki, A., D’Alessio, A. C., Dymov, S., Labonté, B., Szyf, M., Turecki, G., & Meaney, M. J. (2009). Epigenetic regulation of the glucocorticoid receptor in human brain associates with childhood abuse. Nat. Neurosci. 12(3): 342-348.
- Farrell, C., Doolin, K., O’ Leary, N., Jairaj, C., Roddy, D., Tozzi, L., Morris, D., Harkin, A., Frodl, T., Nemoda, Z., Szyf, M., Booij, L., & O’Keane, V. (2018). DNA methylation differences at the glucocorticoid receptor gene in depression are related to functional alterations in hypothalamic-pituitary-adrenal axis activity and to early life emotional abuse. Psychiatry Res. 265: 341-348.
- Checknita, D., Ekström, T. J., Comasco, E., Nilsson, K. W., Tiihonen, J., & Hodgins, S. (2018). Associations of monoamine oxidase A gene first exon methylation with sexual abuse and current depression in women. J. Neural. Transm. 125(7), 1053-1064.
- Cecil C. A., Smith R. G., Walton E., Mill J., McCrory E. J., & Viding E. (2016). Epigenetic signatures of childhood abuse and neglect: Implications for psychiatric vulnerability. J. Psychiatr. Res. 83: 184-194.
- Hein, S., Thomas, T., Yu Naumova, O., Luthar S. S., Grigorenko E. L. (2019). Negative parenting modulates the association between mother’s DNA methylation profiles and adult offspring depression. Dev. Psychobiol. 61(2): 304-310.
- Wanner, N.M., Colwell, M.L., Faulk, C. (2019). The epigenetic legacy of illicit drugs: developmental exposures and late-life phenotypes. Environ. Epigenet. 5(4):dvz022.
- Schrott, R., Murphy. S.K. (2020). Cannabis use and the sperm epigenome: a budding concern? Environ. Epigenet. 6(1):dvaa002.
- Champagne F.A., Francis D.D., Mar A., & Meaney M.J. (2003). Variations in maternal care in the rat as a mediating influence for the effects of environment on development. Physiol. Behav. 79(3): 359-371
- Weaver I.C., Meaney M.J., & Szyf M. (2006). Maternal care effects on the hippocampal transcriptome and anxiety-mediated behaviors in the offspring that are reversible in adulthood. Proc. Natl. Acad. Sci. USA 103: 3480–3485.
- Szyf, M., Weaver, I., & Meaney, M. (2007). Maternal care, the epigenome and phenotypic differences in behavior. Reprod. Toxicol. 24(1): 9-19.
- Felitti, V.J., Anda, R-F., Nordenberg, D., & Williamso, D.F. (1998). Relationship of childhood abuse and household dysfunction to many of the leading causes of death in adults. The adverse childhood experiences (ACE) study. Am. J. Prev. Med. 14(4): 245-258.
- Bouchard, T. J. (2004). Genetic Influence on Human Psychological Traits: A Survey. Curr. Dir. Psychol. Sci. 13(4): 148-151.
- Bueno, D. (2019) Genetics and Learning: How the Genes Influence Educational Attainment. Front. Psychol. 10: 1622.
Annex 1
Heritability of Holland’s Six Personality Traits. Modified from [36].
Six Holland Code | Heritability
(H2) |
Shared
environmental effects |
Realistic | 0.36 | 0.12 |
Investigative | 0.36 | 0.10 |
Artistic | 0.39 | 0.12 |
Social | 0.37 | 0.08 |
Enterprising | 0.31 | 0.11 |
Conventional | 0.38 | 0.11 |
H2 stands for broad sense heritability, which considers al genetic influences. Shared environmental effects are those aspects of an individual’s environment that are necessarily shared with other people in the family, and are distinguished from non-shared ones, which are any aspect of the environment and any experiences that may be different for different children within the same family.
Annexes
Annex 2
Heritability of some traits linked to learning processes. Modified from [37].
Trait | Heritability |
Intelligence | from 0.2 to 0.8, depending on age
0.22 to 0.93, depending on test |
Extremely high intelligence | 0.33 |
Creativity | 0.08 to 0.62, depending on test |
Working memory | 0.39
0.40-0.65 0.72 |
Experimental thinking | 0.44 |
Rational thinking | 0.34 |
Resilience (positive adaptation in the face of adversity) | 0.52 (males)
0.38 (females) |
Emotion-oriented coping (as a strategy used to manage adversity) | 0.14
|
Task-oriented coping (as a strategy used to manage adversity) | 0.11 |
Attention focus | 0.28 |
Cognitive control | 0.49 |
Grit (perseverance) | 0.37 |
Planning ability | 0.53 |
Cooperativity | 0.13 |
Relational processing | 0.67 |
Literacy and Numeracy | 0.68 |
Musicality | from 0.21 to 0.51, depending on test |
Drawing ability | 0.29 |
Exam results
|
0.57 (in mathematics)
0.66 (in humanities) |