Learning and memory: How the brain codes knowledge
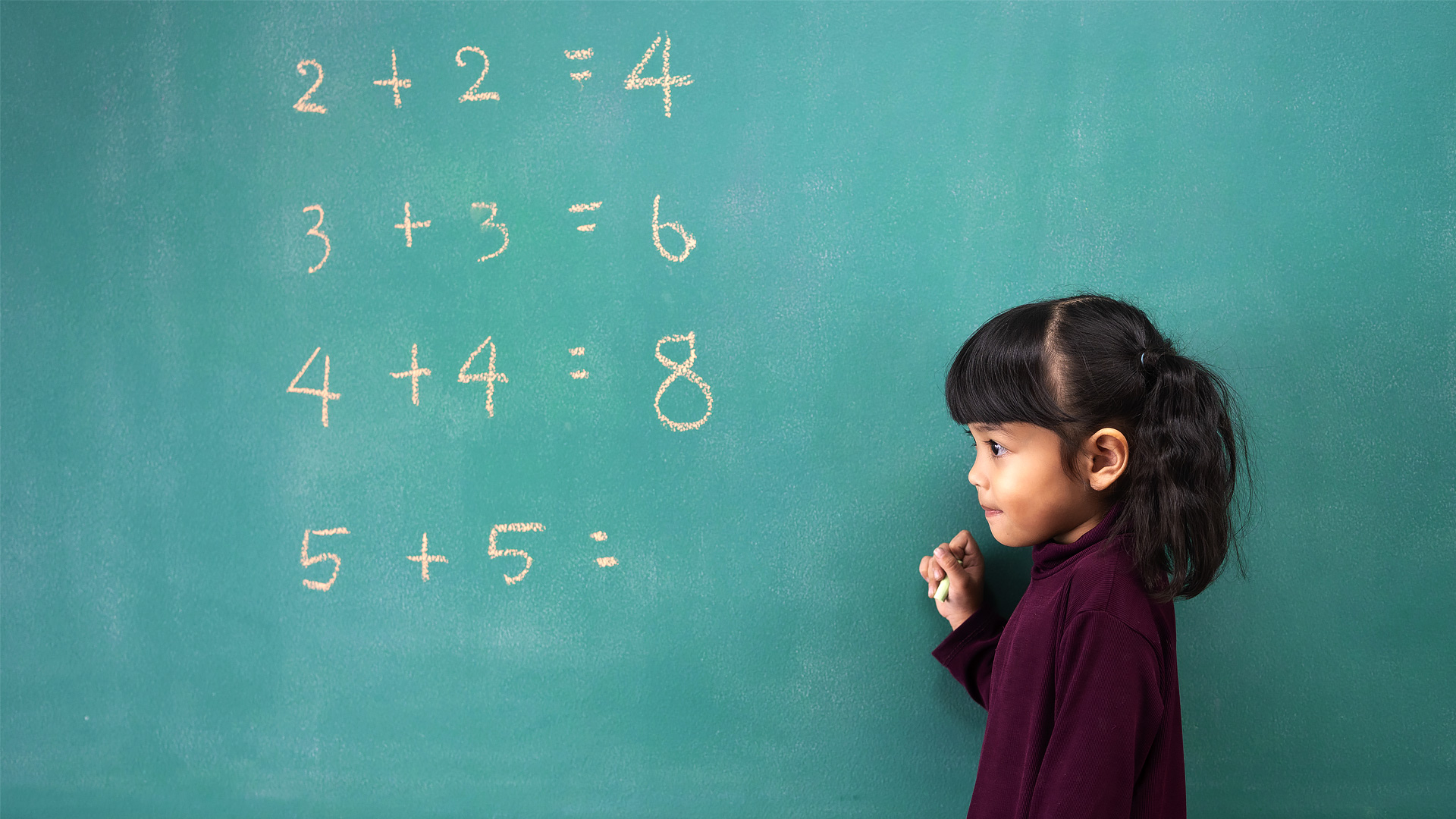
Executive summary
- Learning and memory are necessarily linked in the brain. You have only learnt something when it is coded in some form of memory for recall or use as a skill in the future. Neuroscience considers learning the same as acquiring or strengthening information in memory.
- Memory is not a single thing in the brain. There are different forms of memory for facts, skills, and events of our life that involve different areas distributed across the brain.
- Knowledge is thought to be coded in the brain in an interconnected network, with similar or associated items and concepts being more strongly connected. Recall or retrieval of some fact, memory, or piece of knowledge will spread activation to help recall of other similar and associated memories or knowledge.
- Our brain, therefore, learns by making and strengthening connections between things in memory by association and context.
Learning and memory in the brain
If we define learning broadly as the acquisition of knowledge or skills (either through instruction, study, or experience), then the acquired knowledge or skills must be stored somehow in the brain for recall and use later. This, of course, is the role of memory. Therefore, learning and memory are closely linked in the brain.
Memory is not only a single thing in the brain. Rather, there are several different forms of memory, each with different processes that rely on different areas distributed across the brain. We see this clearly in people who damage particular areas of the brain and have different types of memory loss.
One very famous case, from whom we have learnt an enormous amount about memory and the brain, is a man called Henry Molaison (known as HM)1. HM had severe epilepsy and underwent a radical new surgery in 1953 to remove part of his brain known as the hippocampus. We know that the hippocampus is important for memory and learning, but it was also part of the area that was causing HM’s epilepsy. The surgery was very successful for controlling HM’s epilepsy, but unfortunately left him with very severe memory problems (see Ref. 2 for classic and recent advances in understanding amnesia).
HM’s main problem was that he could not make any new memories, and so he could not remember anything from after the time of his surgery. Even when introduced to someone new, if they were to leave the room and come back a few minutes later, HM would not remember having ever met them before. Every moment in his life after his surgery was new to him.
HM could not learn new words, faces, or facts because he could not form the memories to hold them. Interestingly, HM could recall events and knowledge that he had learnt from before the time of his surgery. This tells us that the processes in the brain for holding and recalling memories or knowledge are separate from the brain processes for learning and acquiring memories or knowledge.
HM could also learn new skills such as writing or tracing shapes in reverse while watching only in a mirror. He would improve day-by-day as he practiced these tasks, although he could never consciously remember having done the tasks before. This tells us that the memory for learning new skills with practice is separate from the memory for learning facts and knowledge for conscious recall.
This gives us several important definitions for different forms of memory. Firstly, we make a distinction between long-term and short-term memory. Short-term or working memory relies on the frontal lobes of the brain and is what we use when keeping things in mind immediately while working on a task or problem. Long-term memory is really what we consider when thinking about learning. It is memory for knowledge or skills that is acquired, stored, and recalled for use sometime in the future and has several important divisions. Refer to Ref. 3 for more information on the differences between short-term and long-term memory.
Knowledge versus skills: Declarative and procedural memory
Long-term memory is divided into declarative memory for facts and knowledge that we can consciously retrieve and “declare,” and procedural memory for unconscious or automatic skills that we acquire such as how to ride a bicycle or how to make the finger movements necessary to form readable characters when writing.
When learning to read, we do not think about how to decipher the patterns of ink on the page (procedural memory), but we do consciously retrieve the meanings of the words (declarative memory). Maths also requires the use of procedural and declarative memory. Number fluency requires understanding of place value and the relationship between operations when solving equations (procedural memory), whereas number facts are basic equations learnt off by heart, such as 3 x 4 = 12 (declarative memory).
Facts versus events: Semantic and episodic memory
Our conscious knowledge, or declarative memory, is further divided into semantic memory for basic facts, meanings of words, and concepts, and episodic memory for recalling life events and episodes such as what we did on our last birthday.
Learning facts (semantic memory) can also be associated with the episode of having learnt the information. Studies have shown that recall of facts is better when retrieval occurs in the same or replicated environment where the content was learnt (or encoded)4,5.
Knowledge coded in the brain: Grandmother cells
With these basic definitions of learning and memory, we can now look at theories about how the brain codes and stores knowledge. The brain is made up of brain cells known as neurons that are massively interconnected through links known as synapses. A single neuron in the brain may be connected to hundreds or thousands of other neurons through its synapses. With about 86 billion neurons in the brain, we estimate that there are 150 trillion or so connections, or synapses. It is this massive network of interconnections that gives the brain its immense computational power.
These connections between neurons through the synapses are continually changing with learning and experience. Learning in the brain is all about making and strengthening connections between brain cells.
So how is knowledge coded in the brain? In the late 1960s, the neuroscientist Jerry Lettvin famously wrote about grandmother cells, neurons in the brain that uniquely represent your grandmother6. These brain cells theoretically fire their signal, communicating to the rest of your brain, whenever you encounter or think of your grandmother, whether in person or photograph, imagined or remembered, or even by name or another abstract form. These cells therefore represent or code the concept that is your grandmother in your brain. If we could somehow find all of the grandmother cells in your brain and remove them, you would completely lose all memory of your grandmother. However, this does not mean that there are cells that do nothing except code for your grandmother.
While this is a quirky example, the theory is that meaningful concepts are uniquely represented or coded in the brain by unique sets of neurons. Since we have billions of neurons within the interconnected network of cells that make up the brain, we can code billions of concepts or memories within this network, each represented by a unique pattern across different sets of neurons.
Scientific evidence for grandmother cells took a long time, but in 2005 a group of researchers managed to measure activity from single neurons in the brains of some patients undergoing surgery for treatment of their epilepsy. What the researchers found were not grandmother cells, but Jennifer Aniston cells—neurons that fired their signal to the rest of the brain specifically when the patient saw pictures of the famous actress Jennifer Aniston! Notably, this network of cells did not respond to pictures of other famous or familiar people, buildings, or objects. However, it is also important to emphasise that when researchers find a neuron firing to a particular concept, this neuron is part of a network of cells. In this context, the network of cells in question specifically fired their signal, communicating to the rest of the brain, only for Jennifer Aniston. This fits with the theory that specific concepts, such as your grandmother or Jennifer Aniston, are coded by unique patterns of activity in different sets of neurons in the brain (refer to Ref. 7 for conceptual clarification).
The importance of connections: Learning by association and context
So we have individual concepts, memories, or pieces of knowledge, each coded in the brain in unique sets of neurons. Importantly, these concepts are linked together in the interconnected network of the brain through the synapses, or connections between neurons. It is these connections via the synapses that are constantly changing in our brain with learning and experience.
Do you have a particular memory or idea that always pops into your head automatically when you see or think about a specific place, person, object, or event in your life? Perhaps there is a particular smell or food or place that always reminds you of your grandmother? This is because of the connections that have formed between your grandmother cells and other cells frequently associated with your grandmother. Encountering one, such as the smell of lavender, will lead to spreading activation to other associated concepts, such as your grandmother, and recollections of your grandmother pop into your mind. It is the connections that code the memory.
Another example, if I ask you what is the capital city of France, you will probably also think of red, white, and blue, cheese, and croissants, or personal memories of events in your life related to France and Paris. Activation will spread to all things that you have personally learned that are associated with France, leading to automatic or easier recall of those associated memories or pieces of knowledge.
The well-known phrase “neurons that fire together wire together” describes learning by making and strengthening connections between associated items, known as Hebbian learning. When two connected neurons fire their signal at the same time, the connection between them is strengthened so that, in the future, activation of one is more likely to lead automatically to activation of the other.
For example, if you encounter the smell of lavender and your grandmother at the same time, your lavender cells and your grandmother cells will form a connection. That connection will be strengthened the more often you encounter those two together. In the future, when the two share a strong connection, activation of one will lead to automatic or easier activation of the other. In psychology, the same idea is known as associative learning, whereby items that are commonly encountered together lead to stronger association so that recall of one leads to automatic or easier recall of the other in the future. This process of making and strengthening connections between neurons that are encountered and activated together is thought to represent most learning in the brain.
In education, this concept can apply from very simple to very complex knowledge. If you ask a preschool child what is a cat, they may say something like “a small animal with fur that says meow,” showing that their concept of cat is encoded by its associated features (animal, fur, meow). Similarly, if you ask a university political science student what is a socialist system of government, they are likely to reply in terms of associated features or concepts.
Knowledge is therefore thought to be coded in the brain by unique patterns of activity across different sets of neurons that are linked and spread activation to other associated concepts, memories, or pieces of knowledge. Learning involves making or strengthening the connections between concepts as they are encountered or recalled together.
So now you have been introduced to grandmother cells and Jennifer Aniston cells, it is likely that your neurons representing Jennifer Aniston and those representing your grandmother are now associated in your brain and share a connection. Perhaps next time you see Jennifer Aniston on the television, you might automatically think of your grandmother!
The importance of recall: Consolidation of learning
By Hebbian learning, neurons firing together wiring together, the recall of associated concepts will also involve activation of the neurons representing those concepts at the same time, further strengthening the connection between them. This has very important implications for recall and consolidation of learning.
For example, if I ask you “What is the name of the theoretical cells that were supported by the discovery of Jennifer Aniston cells in the brain?” hopefully you will recall grandmother cells. Now, again, your neurons representing Jennifer Aniston and those representing your grandmother have been activated together, purely through recall, and the connection between them will be further strengthened.
Similarly, a political science student asked to describe a socialist system of government, and given the opportunity for discussion and feedback with a teacher or peers, will further strengthen the connections between associated concepts, as they are activated together, consolidating their learning.
Implications for education and learning
Two immediate implications of this understanding of learning and memory in the brain are:
- Rote learning of disconnected facts is difficult because it is generally not the way the brain learns. Rather, the brain learns by making and strengthening connections between associated concepts so that learning in context and by linking and association with related knowledge will be more effective. This is seen very clearly in the benefit of using mnemonics that can greatly help learning by creating meaningful associations between items. Using the phrase “never believe a lie” is an example of a mnemonic to remember the correct order of the ‘i’ and the ‘e’ in the word ‘believe.’
- Activities that promote recall or reevaluation of knowledge are highly beneficial for learning by reinforcing and further strengthening the connections between associated items that are recalled together. Examination and assessment should therefore be structured in a way that aids the recall of associated concepts, reinforcing the neural connections between these items and further consolidating learning. In this way, appropriately structured examination can be an integral part of learning itself. How much should we facilitate recall during an exam and how much should we challenge it by not giving clues?
References
- Squire, L. The legacy of Patient H.M. for neuroscience. Neuron 61, 6-9 (2009).
- Allen, R. Classic and recent advances in understanding amnesia. F1000Research 7, 331 (2018).
- Norris, D. Short-term memory and long-term memory are still different. Psychological Bulletin 143, 992-1009 (2017).
- deBettencourt, M., Turk-Browne, N. & Norman, K. Neurofeedback helps to reveal a relationship between context reinstatement and memory retrieval. NeuroImage 200, 292-301 (2018).
- Smith, S. A comparison of two techniques for reducing context-dependent forgetting. Memory & Cognition 12, 477-482 (1984).
- Gross, C. Genealogy of the “Grandmother Cell.” The Neuroscientist 8, 512-518 (2002).
- Quian Quiroga, R. & Kreiman, G. Postscript: About grandmother cells and Jennifer Aniston neurons. Psychological Review 117, 297-299 (2010).