Brain development and its derangements
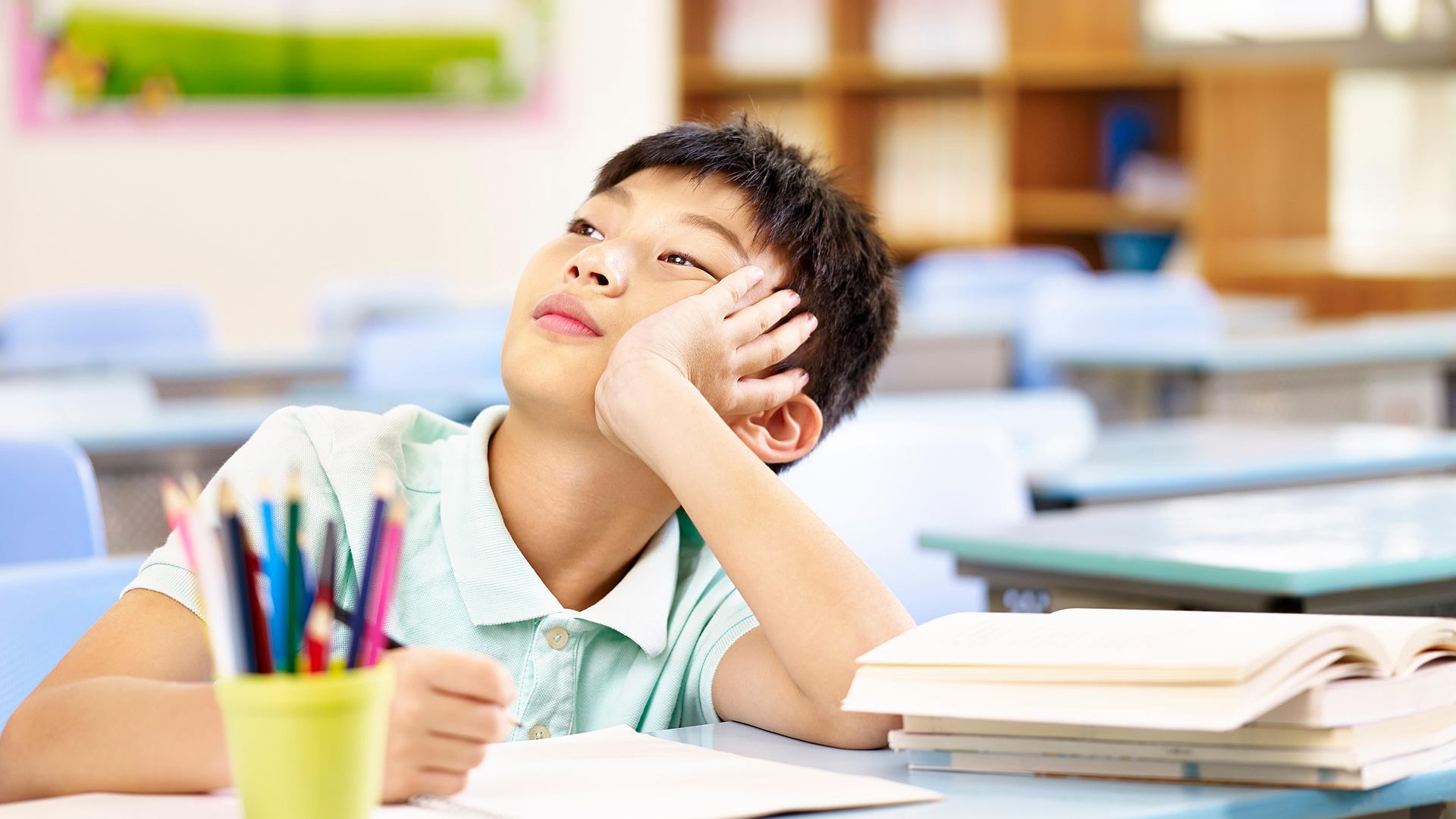
Executive summary
Neuroscience has unraveled a great part of the developmental phenomena, at least in descriptive terms. Correlations between the different developmental phases and the emergence of behavior and mental features of children, however, lag behind, given the difficulty in assessing them in utero and even during the first phases of postnatal life. During development after birth, some periods are more sensitive to the influence of environment, and they become optimal targets for educational interventions. Despite the route established by nature, and the modulation by nurture, things may go wrong and originate developmental disorders that may impact negatively in the education of atypical children. Neuroscience, psychology, and pedagogy can be targeted to fix as well as possible the disarrangements of development.
From the encounter of two smart cells to the birth of a human being
Everything begins at the beginning, a philosopher of the obvious would say. But…when is the beginning of human life? In fact, this is not an obvious issue, rather one of the most controversial debates of current times, involving scientists, philosophers, and jurists: When does life of an individual begin? A scientific answer might be that life never begins, it continues ever. This is because the sperm and the ovule are living cells, which fuse together to transmit their life to the fertilized egg that will develop into a new human being.
An equally controversial issue, which still lacks a convincing resolution even now, relates to the beginning of human consciousness. Does the fetus feel, perceive? Does it think something? If so, when does this ability start? As we will see, there is evidence that human fetuses do indeed start to show sensory responses around the 18th week of gestation, but how much this suggests “consciousness” is still unknown1.
After fertilization, the egg moves through one of the uterine horns, and along its way it divides into 2, 4, 8 cells and so on, forming a minuscule sphere called morula. A cavity then starts to form within this sphere, which falls into the uterus, adheres, and encrusts as a true cyst. The cyst will now multiply its cells to give rise to the embryonic layers that will form the fetus. There is no consciousness at this point, because there is no nervous system yet.
Within the uterine wall, the embryonic spherule grows continuously, until one of its germ layers—the ectoderm—gives rise to the first sign of a nervous system around the third week of gestation, in the form of a plate. The neural plate then folds in on itself and forms a cylindric tube, and the tube convolutes as a water hose, to finally form the first stage of the central nervous system (see Figure 1). One difference between this folded structure and a hose is that the cranial part at one end of it (which will become the brain) proliferates so much that it becomes thicker and bigger than its bodily part (which will be the spinal cord). Even full of folds, the embryonic nervous system is still a tube, with an internal cavity delimited by a wall of cells.
The cells from the internal wall of this convoluted tube are progenitors, that is, they will give origin to neurons and other cells in the brain. They initially form a single layer and generate other progenitors through successive cycles. But, at a certain moment, the daughter cells stop dividing and stabilize as newborn neurons. Neurons “are born” and do not divide anymore. They die during our life, or with us when we die. The production of new neurons in the developing human brain (neurogenesis) can reach the gigantic speed of 250 thousand cells per minute! Neurogenesis is an important phenomenon because, although it persists in adults only within some specific brain niches, it is regulated very powerfully by the environment2, for the good or for the evil, being a known mechanism of neuroplasticity3.
The first neurons are born around the 10th gestation week (see Figure 1) and migrate to form the layers of the cerebral cortex and the nuclei of subcortical regions—a process that continues until very near the birth of the baby. When anomalies take place during these events, interrupting or changing proliferation and/or neuronal migration, severe malformations may produce heavy consequences for the child. This is the case of some infections such as that of the Zika virus, which cause microcephaly4—the baby is born with a very small brain, and severe retardation follows during postnatal development. During migration, young neurons sprout and extend fibers from the cell body—many dendrites and one axon. The dendrites will be the antennae that receive information from other neurons at junctions called synapses, while the axon will be the output cable of each neuron, in charge of conveying information to the next neuron, after it is processed by its cell body membrane.

Figure 1. Timeline of brain development (center) in parallel with cognitive abilities (left, in green) and with the main neurobiological phenomena (right, in blue). The embryo enlarged at lower left shows the three basic brain parts of the primitive brain (asterisks) starting to convolute. Modified from Lent (2019), The Apprentice Brain5 (illustration by Julio Xerfan).
Since these developmental phenomena will take place with all the 86 billion neurons in the human brain, the result will be the amazing set of one thousand trillion circuits we have. It is probable that these gigantic numbers are achieved shortly after birth. Synapses are the sites of the computations the brain can perform and, since they may be strengthened or weakened as a result of memory and forgetting, they are considered crucial for learning and education. Synaptogenesis (the birth of connections) starts at about the 20th gestation week and extends across birth until adolescence. It does not disappear, however, but just declines in adulthood and ageing.
As soon as the neural hardware becomes established, the software starts to work as well. Thus, the molecular machinery that allows the production of electrical impulses by nerve cells ripens, and neurons start to communicate actively. Brain areas, therefore, start to establish contact through functional networks that can be recorded while the baby is still in utero6.
As mentioned, an important, though undefined, issue—with great social, ethical, and legal repercussions—is whether the fetus displays any kind of “consciousness” before birth, or even any kind of sensory perception such as hearing and touch. The possibility of detecting pain perception in the fetus is obviously of great relevance to pediatric medicine and developmental psychology.
Some mothers report noticing that their baby, as soon as they are born, stops crying and looks attentively to her or to daddy as if recognizing their voices, even amid the noises in the birth room. This kind of incidental observation is supported by scientific evidence. By recording changes in cardiac rhythm as attention markers, it has been shown that newborns are able to recognize their mom’s voice from that of other women in the room and, after going home, they show more interest in stories that were told by mom during gestation. This kind of differentiated perception of maternal voice can be detected by functional brain imaging even before birth7. The baby cannot understand the language and the story, of course, but this shows the surprisingly precocious potential of an infant—even before birth—to interact neuropsychologically with the world around.
Sensitive periods: Windows of opportunity for learning
Time is an essential variable in development. With time, however, changes in the nervous system and their cognitive correlates are not linear, but have the shape of an inverted U. They increase up to a peak and decline afterwards, and so does the susceptibility of the nervous system to environmental modulation. This susceptibility is known as neuroplasticity. The higher levels of neuroplasticity mean that there are periods during development when improvement of a given ability or some kind of learning takes place faster and easier. These “windows of opportunity” of the brain are called sensitive or critical periods (see Figure 2).
Sensitive periods must be understood as intervals during which the mechanisms of brain plasticity are especially active and prone to receive the appropriate stimulation from the environment. Sensitive periods have been identified for complex neuropsychological phenomena as imprinting, phonetic learning, music learning, and extinction of fear memory. Sensitive periods have also been identified for sensory abilities such as binocular perception and spatial localization of sounds.
Along childhood and adolescence, different aspects of cognitive and social emotional functions undergo periods like these. Concerning language, for instance, there is evidence of multiple sensitive periods with different start points and durations. A series of studies with children during the first postnatal year showed that, at first, they are able to discern phonemes not only of the languages they hear daily, but also from languages they have never heard before8. After one year old, this ability disappears and the child is able to identify only the phonemes of their main languages.
During this early period, babies already perceive simple musical rhythms (that of a march, for instance), although they do not do so well for other rhythms (that of a waltz, for example)9. Other aspects of language seem to have different sensitive periods. The one for syntax learning, for instance, has been identified between 18 and 36 months postnatal. Vocabulary, on the other hand, shows great achievements at 18 months, and continues to be improved along the whole life.

Figure 2. Each aspect of development has its own sensitive period (A), represented as an inverted-U curve. The exact chronology of each sensitive period is poorly known. Besides, it probably varies with different children, producing different speeds of psychological development and, sometimes, developmental disarrays (B). A change of sensitive periods, for example, is one of the hypotheses to explain the origins of autism. Modified from Lent (2019), The Apprentice Brain5 (illustration by Julio Xerfan).
What about adolescence? This is defined as a transition period between childhood and adulthood, and it is during this period that puberty takes place, the phase in which various endocrine glands mature, impacting on the secondary sexual organs and changing the child’s body and mind gradually into an adult one. The “hormonal explosion” of adolescence occurs for many endocrine glands, under control of a brain region called the hypothalamus, responsible for the maintenance and regulation of the functional balance of the body. The hypothalamus is connected to the pituitary both by its own hormones and by nerve fibers. It is the hypophysis under hypothalamic control that acts at a distance in different targets of the body by hormones secreted into the blood.
The sensitive period of adolescence includes also the completion of development of the prefrontal cortex and its connections with the hippocampus and the so-called dopaminergic reward system. These are components of a circuit essential for control of executive functions (response inhibition, interference suppression, and performance self-regulation), and for the optimal operation of working memory, responsible for the continuity of the present and its connection with the immediate past. Because of their relative brain immaturity, adolescents also display great activity of the central reward system and of those regions responsible for attributing emotional valence to external stimuli. This characteristic makes them more prone to accept challenges and take risks.
Adolescence is the final stage of both physiological and neuropsychological maturation of human beings, who now have their brains heading to adulthood. It is the definitive transition to our neuropsychological profile, over which adult neuroplasticity is going to operate, employing mechanisms quite different from those operating during childhood.
When things go wrong during development
When things go wrong during development, we say the child presents with a developmental disorder. Among the most frequent are attention deficit and hyperactivity disorder (ADHD), the specific disorders of learning (dyslexia and dyscalculia being the main examples), and autism (autism spectrum disorders). Let us describe briefly what these disorders are, as examples of developmental derangements.
Children with ADHD are sometimes identified by parents and teachers, but since inattention may have different causes, the diagnosis must be done by a child psychologist or psychiatrist. The child is typically incapable of focusing attention on one task and concentrating on it for a reasonable amount of time. He/she changes focus many times and therefore tasks are never completed. Performance in school, of course, is highly impaired. In addition, hyperactivity and impulsivity are the rule, with frequent and intensive body movements, inappropriate social behavior, and excessive talking. Since these characteristics are not all-or-none, the exact cutoff point between typicality and disorder is difficult to establish.
What happens in the brain of children with ADHD? Not much is known about that, but evidence suggests a dysfunction of the dopaminergic reward system10. This is a set of regions in the brain, characterized by mediation by the neurotransmitter dopamine, which becomes active not only when a reward is obtained after a certain behavior, but also when the anticipation of the reward is perceived. Children learn to pay attention during class to succeed in the exams. When they succeed, teachers and parents greet them, and they feel proud of their achievement. This is the reward, while the class work period represents an anticipation of the reward. It has been shown that the reward regions in the brains of typical children become active not only when reward arrives, but also before it, during anticipation of its arrival. This is not so for children diagnosed with ADHD. The brain, in this case, only becomes active with the reward itself, but fails to function during the anticipation period. As a result, the child abandons his/her work and seeks something else to do. Other evidence favors a genetic cause for this condition, of complex, multigenic determination. The possible changes during brain development that give rise to these differences are still poorly known.
Specific learning disorders are equally very difficult to approach scientifically, in terms of their causes and mechanisms. This is because employing animal models is insufficient as means to provide mechanistic explanations. Reading and mathematics are products of culture. They represent, therefore, adaptations of the biological configuration of the brain that substitute functions selected by evolution for others created by human culture. Noticeably, the first pieces of evidence for a defective morphology of neurons and neuronal groupings in the cerebral cortex of dyslexics were produced decades ago11. Given the nature of the defects, the best interpretation was that of irregularities in the migratory behavior of neurons of cortical areas related to speech and reading during development.
The same kind of defect in neuronal migration during brain development has been proposed more recently for autism spectrum disorders12. Autistic children have difficulties in social communication and interaction with others and, perhaps because of this, they adopt repetitive patterns of behavior and have few interests and activities. It is believed that they do not develop the cognitive ability known as theory of mind, which means not an intellectual theory, but rather a hypothesis one makes about another’s mental status by interpreting his/her facial expressions and body gestures, language intonations, and other emotional signals implicit in behavior.
Despite the lack of a solid, mechanistic explanation of these disorders, it is clear that they result from anomalies in brain development. By using modern imaging techniques, neuroscientists are working on revealing the changes in regional brain activity in these conditions. A very recent alternative to the search of mechanisms in the living human brain is the use of minibrains, cultivated from induced pluripotent stem cells taken from the skin or urine of autistic individuals and other participants13.
Nature shapes nurture along brain development
Neurodevelopment is a very strategic opportunity in biology to observe the way nature and nurture interact to sculpt the human mind and behavior. Brain development occurs through interaction of the environment and our genes, which are characteristic of our species, and a result of evolution. Thus, scientists are able to reveal the general laws and principles that govern development. This means that a general sequence of events can be described which predicts the final result: a human being able to learn, speak, display complex motor skills, perceive the world through the senses with an exquisite acuity, and construct a cultural apparatus that characterizes civilization. At the same time, human individuals are very different, both physically and mentally. There is no human who is exactly the same as another, even considering identical twins. It is unescapable, therefore, that human development is individually modulated by the environment. And in this case, education plays an utmost important role because it is a structured way to direct the influence of the environment on development, or, more generally, the influence of nurture on nature.
- Borsani, E.; Della Vedoca, A.M.; Rezzani, R.; Rodella, L.F.; Cristini, C., Correlation between human nervous system development and acquisition of fetal skills: An overview. Brain & Development 41:225-233 (2019).
- Gomes da Silva, S. et al. Maternal exercise during pregnancy increases BDNF levels and cell numbers in the hippocampal formation but not in the cerebral cortex of adult rat offspring. PLoS ONE 11(1):e0147200 (2016).
- Leal-Galicia, P.; Romo-Parra, H.; Rodríguez-Serrano, L.M.; Buenrostro-Jáuregui, M., Regulation of adult hippocampal neurogenesis exerted by sexual, cognitive and physical activity: An update. Journal of Chemical Neuroanatomy 101:101667 (2019).
- Garcez, P.P. et al. Zika virus impairs growth in human neurospheres and brain organoids. Science 352:816-818 (2016).
- Lent, R., The Apprentice Brain (in Portuguese), Atheneu, Rio de Janeiro (2019).
- Seshamani, S. et al. Detecting default mode networks in utero by integrated 4D fMRI reconstruction and analysis. Human Brain Mapping 37:4158-4178 (2016).
- Jardri, R. et al. Assessing fetal response to maternal speech using a noninvasive functional brain imaging technique. International Journal of Developmental Neuroscience 30:159-161 (2012).
- Kuhl, P.; Ramirez, N.F., Neuroscience and education: How early brain development affects school. In: Developing Minds in the Digital Age (Kuhl, P. et al., eds.), pp. 25-37, OECD (2019).
- Zhao, T.C.; Kuhl, P. Effects of enriched auditory experience on infants’ speech perception during the first year of life. Prospects (Unesco) 46:235-247 (2016).
- Furukawa, E. et al. Abnormal striatal BOLD responses to reward anticipation and reward delivery in ADHD. PLoS ONE 9(2) e89129 (2014).
- Galaburda, A.M.; Sherman, G.F.; Rosen, G.D.; Aboitiz, F.; Geshwind, N., Developmental dyslexia: four consecutive patients with cortical anomalies. Annals of Neurology 18:222-233 (1985).
- Pan, Y.H.; Wu, N.; Yuan, X.B., Toward a better understanding of neuronal migration deficits in autism spectrum disorders. Frontiers in Cell and Developmental Biology 7:205 (2019).
- Setia, H.; Muotri, A.R., Brain organoids as a model system for human neurodevelopment and disease. Seminars in Cell and Developmental Biology S1084-9521(18)30061-2 (2019).