Neuroplasticity: How the brain changes with learning
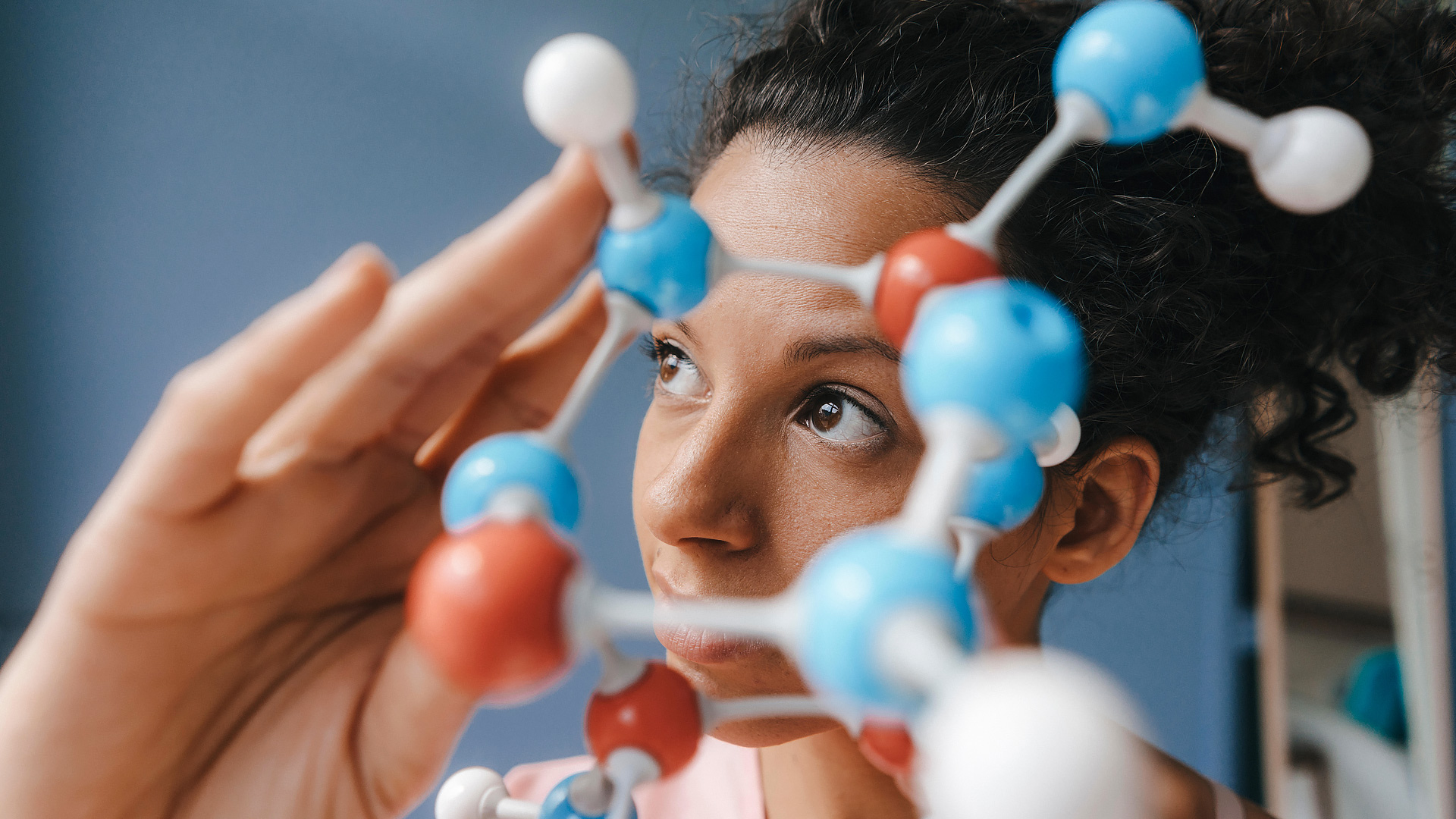
Executive summary
- Your brain is never fixed but continues to change with learning and experience throughout your life.
- Most learning in the brain involves rewiring or making and strengthening connections between neurons, the cells of your brain most crucial for learning.
- In most regions of the brain, the only neurons you will have throughout all of your life are already present at birth. Your brain does continue to grow new neurons in at least one very small but potentially important area for learning, but this is very new research and the role of these new neurons for learning is not yet known.
- Neuroplasticity is important for all learning—much of the neuroscience research on neuroplasticity is related to how the brain recovers from injury or damage—but some of the same principles apply to how the brain changes with learning throughout all of life.
Learning and memory and changes in the brain
Let us start with a simple logical argument, to dispel myths and show that logically, of course, your brain is changing with learning:
Learning and memory are necessarily closely linked. You cannot learn something without storing it in some form of memory for use in the future, either for recall as new knowledge or improvement in skills. From neuroscience, we know that memories are encoded by physical changes in the brain (although we still debate exactly what it is that changes and how). Your brain therefore changes physically whenever anything is learnt, and so your experiences and learning throughout all of life change and mould your brain.
There is a common brain myth or popular perception that the brain becomes fully developed sometime in early childhood, implying that nothing further changes, and that further changes in the brain with development in childhood and adolescence are somehow biologically determined, leading to a fully developed state at adulthood.
In reality, the way your brain develops is determined both by your genetics and by your learning and experiences. Biological does not mean predetermined. Your brain is shaped by your experiences and is never fixed but continues to change along with learning across the lifespan.
Some neuroscience history—the structure of the brain and the origin of myths
In the late 19th century, the biologist Ramón y Cajal proposed the neuron theory of the brain, that the brain is composed of discrete but interconnected cells, similar to the cells that make up the rest of the body1. He was ridiculed by his contemporaries who believed that the brain was made of an entirely different type of matter than the rest of the body, but further research confirmed Cajal’s theories and in 1906 he received the Nobel Prize for his neuron theory.
The brain, of course, is made of cells known as neurons that are densely interconnected through links known as synapses. Most neurons of the brain are found in the outer surface layer that is only a few millimetres thick, known as the grey matter or cortex. The highly folded surface layer of the brain, that gives the brain its characteristic bumpy appearance, maximises the amount of grey matter and therefore the number of neurons that can be packed into the brain to still fit within a relatively small skull.
One thing that Ramón y Cajal got drastically wrong, however, was his claim that: “In adult centres the nerve paths are something fixed, ended, immutable. Everything may die, nothing may be regenerated2.” He was partly correct, in that neurons will never repair or regenerate when they are damaged. Damaged areas of the brain or damaged cells in a broken spinal cord, for example, will never heal by regrowing or regenerating new cells.
However, this has led to the myth that the brain reaches some point in early adulthood after which it never changes, except to go downhill as we age! The brain is never “fixed, ended, and immutable,” but continues to change with learning throughout all of life. It does this mainly by changing the wiring or the interconnections between neurons.
In a quirky study, London taxi drivers show that humans have a remarkable capacity to acquire and use knowledge to navigate their complex city without a map. This wiring is reflected by their particularly large hippocampus3.

Figure 1. Exquisitely detailed hand-drawings by Ramón y Cajal (1899)4 show the main cells of the brain (neurons) and their extensive interconnections (via synapses) in the surface layer (grey matter) that makes the true structure of the brain.
The importance of brain connections—synaptogenesis
It is the vast network of interconnections between the neurons of the brain, through links known as synapses, that gives the brain its immense computational power. While the brain contains an enormous number of neurons, about 86 billion, each of those neurons can be connected to many thousands of other neurons, giving an estimated 150 trillion or so connections or synapses in the brain.
The vast majority of our neurons are located in the surface grey-matter layer of our brain. The number of neurons we have does not change with learning or life experience.
The connections between neurons, through the synapses, however, are constantly changing throughout all of our life and are predominantly responsible for learning and memory in the brain. These changes in connections involve forming new connections, known as synaptogenesis, or strengthening existing connections, known as long-term potentiation (LTP).
Much of what we know about synaptogenesis comes from studies of animals raised in highly stimulating environments (similar to a rat’s natural environment) compared with those raised in deprived environments. A prominent neuroscientist of the 1940s, Donald Hebb, first showed how rats raised as pets performed better on many cognitive tasks than rats raised in laboratory cages. When rats were provided adequate opportunities for physical, social, and sensory stimulation, more extensive interconnections between their neurons can form, with a greater number of synapses5.
We assume that the same processes are important in human brains but have little direct evidence. Studies conducted with children from extremely deprived situations in Romanian orphanages of the 1980s show persistent delays in cognitive, language, and social development, so we know that severe deprivation is detrimental6. Interestingly, recent research on Albert Einstein’s brain has suggested that he had a more connected brain, with more dense neuron connections between the left and right hemispheres of his brain, presumably allowing more efficient brain communication7.
Donald Hebb described an important process for learning in the brain, known as Hebbian learning (1949), summed up by the phrase, “neurons that fire together wire together8.”Put simply, when two or more neurons respond or fire at the same time (i.e., from some thought, action, or event in the environment) the connection or synapse between them is strengthened, leading to a stronger association. This means that if some situation (or thought or action) is encountered in the future causing one of those neurons to respond, it will now be more likely to trigger a response in the other connected neurons, recalling and further reinforcing that association.
In this way, much learning in the brain involves changing the connections between neurons, particularly reinforcing those pathways or circuits of interconnected neurons that are used frequently and fire together.
The debate of new neurons—neurogenesis
The vast majority of our brain contains only neurons that have been present since our birth; however, one small but very important area of our brain continues to grow new neurons throughout all of our life, through a process known as neurogenesis. This area is called the hippocampus and is known to play a crucial role in memory and learning.
It is only in the last decade that researchers have shown that new neurons are born in the hippocampus in the human brain throughout life. In the most conclusive study, researchers used a carbon-dating technique to accurately determine the age of individual cells within the hippocampus. They estimated that around 700 new neurons are added to each hippocampus (left and right) every day, and by the time we are 60 years old about one-third of the neurons in our hippocampus will be new neurons formed by neurogenesis after birth9.
This has caused much excitement and is a topic currently at the forefront of research in neuroscience, but there is still a lot we do not know. For example, research only indicates the potential role of the new neurons in the hippocampus in learning or memory10.
We know that the hippocampus overall is crucial for laying down new memories, because people with damage to their hippocampus suffer severe amnesia and cannot remember anything after the time of the damage. We also know that the hippocampus plays a role in spatial navigation, or our ability to remember and “feel” our way around a familiar place, a discovery that led to award of the Nobel Prize in 201411 (refer to https://www.news-medical.net/health/Hippocampus-Functions.aspx).
We know many things that can boost neurogenesis, such as exercise, diet, reducing stress, and learning itself. There are now many books, internet sites, and emerging products advising how to enhance neurogenesis and thereby “boost your brain.” However, in truth we do not know whether specifically targeting neurogenesis is necessary or even beneficial for cognition, memory, or learning and so there is not enough evidence to claim that any of these things will actually “boost your brain.”
What we know is that the hippocampus continues to grow new neurons throughout life and that the hippocampus is crucial for learning and memory. But will enhancing the growth of new neurons in your hippocampus make you smarter? That is for future research to tell.
Neuroplasticity in action
The brain has an incredible capacity to reorganise itself through rewiring, altering, and strengthening the connections and pathways that are used often. Just as Donald Hebb described, those pathways of interconnected neurons that are trained or used frequently, firing together, strengthen their connections and thereby wire together.
Most research on large-scale brain changes with neuroplasticity focuses on how the brain recovers or reorganises following damage or injury. For example, the parts of our brain that control our body movements and our sense of touch have a kind of map of the body, known as a homunculus, so that neurons in a particular area connect to muscles in a specific part of the body. If someone has damage to this motor area of their brain, for example caused by a stroke or blockage of blood supply, then they will have severe weakness with movements of the part of their body corresponding to the part of their brain that is damaged.
As we know, damaged neurons do not heal or regenerate and new neurons never grow in this part of the brain, but nonetheless people can regain control of their movements. With rehabilitation and repeated training of the weak movements, undamaged areas of the brain can remap their connections to take over function from the damaged areas. This is the basis of physiotherapy for movement rehabilitation, creating and strengthening new pathways as the brain relearns to control movement through new connections.
The principles of creating and strengthening connections to mould the brain can also be applied to normal learning, not just the relearning involved following brain damage.
For example, an interesting study showed that people who play string instruments actually have a larger region of the sensory area of their brain devoted to touch sensation of their left hand when compared with their right hand or compared with people who do not play string instruments12. It seems that their extensive practice with finger movements on the strings with their left hand moulds their brain, creating and strengthening connections, so that more of the sensory area of their brain area is connected to their left hand.
In another study, when a group of young adults were taught to juggle and practiced for three months, a particular part of the grey matter of their brain increased in size, in an area important for the perception of moving objects13. When they stopped juggling and were examined after another three months, that area had returned back to its original size. We know that new neurons do not grow in that part of the brain, so the increase in size is not from the brain growing new neurons. A subsequent study showed that connections into the brain’s grey matter changed as people learnt to juggle, suggesting that practice and use of the skill enhances brain connections.
The brain therefore has an enormous potential to adapt and change by altering and strengthening connections through use and experience. The use of particular brain pathways strengthens those pathways. This is thought to be the major way that the brain learns, by adapting and changing connections with experience.
The story of Einstein’s brain
When Albert Einstein died in 1955 his brain was removed during autopsy, photographed, and then dissected into many parts and preserved. There is a long history about what happened with those preserved parts and many sections are still unaccounted for; however, in 2010 a collection of original photographs were uncovered and acquired by the National Museum of Health and Medicine, Washington, DC, USA.

Figure 2. A photograph of Albert Einstein’s brain taken during autopsy in 1955. The collection of photographs taken at that time were lost for many years, but were recovered in 2010 and have been used in recent research to examine what was special about the structure of his brain.
A very recent study has used those original photographs to analyse the density of neuron connections between the left and right hemispheres in Einstein’s brain, through a structure known as the corpus callosum. The researchers compared Einstein’s corpus callosum from the photographs to MRI scans from people of the same age as Einstein when he died (76 years old) and people of Einstein’s age during his so-called miracle year in 1905 when he published four of his groundbreaking scientific papers (26 years old).
Overall, the researchers found that Einstein’s corpus callosum was thicker in most parts than both the elderly and younger comparison groups. This suggests that Einstein had more extensive neural connections between the left and right hemispheres in his brain. The researchers concluded that Einstein’s intellectual gifts may have involved more coordinated communication between the two hemispheres.
Implications for education
These are the basic principles in neuroscience for change in the brain with learning. There is still an enormous gap in explaining how these principles apply to the much more complex situation of schools and education and the process of acquiring knowledge and skills such as reading and mathematics. Nonetheless, these basic principles of neurons and synapses, changing and strengthening connections, are at the root of all learning in the brain.
Some immediate implications to come from these basic principles are:
- The brain always has the capacity to learn and is not predetermined by biology. The brain continues to change constantly with learning and experience throughout all of life and never becomes fixed and unchanging. Therefore, one’s “smartness” is as much about brain connectivity from learning, not just about your biology.
- Learning is essentially something that the brain just does automatically. As we experience different situations in our everyday life, carry out our day-to-day tasks, encounter problems and find solutions, those pathways used in our brain most frequently strengthen their connections, forming habits, reinforcing memories, and improving skills. Of course, learning can also be deliberate, when we practice or train, but the same principles of changing and strengthening connections apply whenever those pathways are used.
- It is recommended that deliberate learning involves making and strengthening connections between associated concepts, rather than the rote learning of disconnected facts.
References
- Cajal, S. Estructura de los centros nerviosos de las aves. 1-10 (Jiménez y Molina, 1888).
- Colucci-D’Amato, L., Bonavita, V. & di Porzio, U. The end of the central dogma of neurobiology: stem cells and neurogenesis in adult CNS. Neurological Sciences 27, 266-270 (2006).
- Maguire, E., Woollett, K. & Spiers, H. London taxi drivers and bus drivers: A structural MRI and neuropsychological analysis. Hippocampus 16, 1091-1101 (2006).
- Cajal, S. Comparative study of the sensory areas of the human cortex. (Clark University, 2013).
- Hebb, D. Committee on Graduate and Professional Training. American Psychologist 2, 206-206 (1947).
- Blakemore, S. & Frith, U. Learning Brain. 32-32 (Wiley, 2005).
- Men, W. et al. The corpus callosum of Albert Einstein‘s brain: another clue to his high intelligence? Brain 137, e268-e268 (2014).
- Cajal, S. Comparative study of the sensory areas of the human cortex. (Clark University, 1899).
- Spalding, K. et al. Dynamics of hippocampal neurogenesis in adult humans. Cell 153, 1219-1227 (2013).
- Lieberwirth, C., Pan, Y., Liu, Y., Zhang, Z. & Wang, Z. Hippocampal adult neurogenesis: Its regulation and potential role in spatial learning and memory. Brain Research 1644, 127-140 (2016).
- Dr. Ananya Mandal, M. Hippocampus Functions. News-Medical.net (2019). at <https://www.news-medical.net/health/Hippocampus-Functions.aspx>
- Elbert, T., Pantev, C., Wienbruch, C., Rockstroh, B. & Taub, E. Increased cortical representation of the fingers of the left hand in string players. Science 270, 305-307 (1995).
- Draganski, B. et al. Changes in grey matter induced by training. Nature 427, 311-312 (2004).